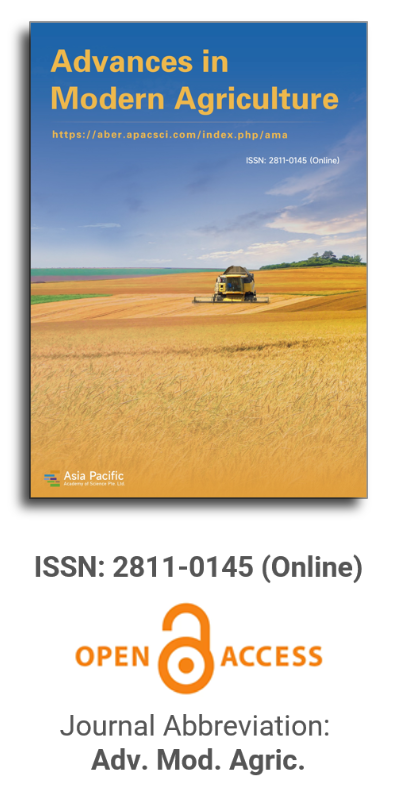
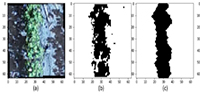
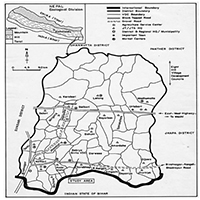
Fluorescence tracer method for analysis of droplet deposition pattern characteristics of the sprays applied via unmanned aerial vehicle
Vol 2, Issue 1, 2021
VIEWS - 4117 (Abstract)
Download PDF
Abstract
With the development of agricultural aviation technologies and their application in agricultural production, unmanned aerial vehicles (UAV) have been widely used to control pests and diseases of crops. The high-speed rotation of the rotor in the UAV produces a powerful downwash, affecting the distribution of pesticide droplets on the ground. Understanding the spatial distribution of these droplets on the ground is important to evaluate the application quality of the pesticides and plays an important role in improving the spray system in the UAV and optimizing its operating parameters. Current methods for measuring the droplet deposition distributions use a number of collectors placed regularly on the ground to receive the droplets and measure their sizes; it is difficult for them to effectively obtain the deposition of all droplets due to the downwash of the UAV. This paper presents a new method to resolve this problem by improving the accuracy and spatial continuity of pesticide droplet measurement applied by an unmanned helicopter. The flying parameters of a 3WQF-80-10 unmanned helicopter used to spray pesticides were obtained from the high-precision Beidou navigation system, and the RQT-C-3 fluorescent whitening tracer with a mass fraction of 1.0% was used as the proxy for the pesticides. Two droplet collection methods—one using continuous strip paper and the other using individual water-sensitive paper—were used to measure the droplet deposition distribution. We divided the experimental field into three areas, with Areas 1 and 2 spaced 3 m apart and Areas 2 and 3 spaced 1m apart. A metal bracket 8 m long and 0.5 m away from the ground was placed in each area. Prior to the experiment, a piece of paper tape was fixed to the surface of the bracket, and the water-sensitive paper cards were placed evenly in the area 0.5 m away from the paper tape. There was one piece of paper tape and 15 water-sensitive papers in each area, and a total of six spray tests were performed based on the pro-designed flight parameters. The combinations of flight speed and flight height were: 2 m/s and 3 m, 2 m/s and 6 m, 2 m/s and 9 m, 3 m/s and 3 m, 3 m/s and 6 m, and 4 m/s and 9 m. The paper tape was detected by fluorescence spectroscopy analysis, and the water-sensitive papers were scanned using image processing software to obtain droplet deposition coverage rates. The results showed that the distribution curves of the coverage rate obtained by the paper tape method coupled with the fluorescence spectrum tracer were consistent with those obtained from the images of the water-sensitive paper method, with the R2 being 0.88–0.96. Because not all fine droplets fell on the water-sensitive papers due to the effect of the high-speed rotating rotor, the coverage rate curve measured by the continuous fluorescence method had multiple peaks, and the value of its coverage rate was higher than that measured by the water-sensitive paper method. When the unmanned helicopter flew at a speed of 2 m/s and a height of 3 m, the coverage ratio obtained from the continuous fluorescence method was up 16.92% compared to that sampled from the individual water-sensitive paper method, while when the flight speed was 4 m/s at a height of 9 m, the coverage ratio in the latter was 97.77% higher than in the former. In terms of the impacts of unmanned helicopter operating conditions on coverage rate, when the helicopter flew at 2 m/s and a height of 3 m, the coverage rate of the droplets obtained from the two methods was the highest, being 8.34% for the continuous fluorescence method and 7.14% for the individual paper method. As the flight height and speed increased, the spatial coverage rate of the droplets decreased. In summary, the high-speed rotor of the UAV generates a downwash, making the droplets of pesticides move in different directions and resulting in a large spatial difference in their deposition on the ground. Therefore, the continuous sampling method is more adequate to evaluate the spatial distribution of the droplets. This study has implications for the study of detecting the deposition of pesticides and other agrochemicals applied by UAVs.
Keywords
References
1. Zhou Z, Zang Y, Luo X, et al. Technology innovation development strategy on agricultural aviation industry for plant protection in China. Transactions of the Chinese Society of Agricultural Engineering (Transactions of the CSAE) 2013; 29(24): 1–10.
2. Zhang D, Lan Y, Chen L, et al. Current status and future trends of agricultural aerial spraying technology in China. Transactions of the Chinese Society for Agricultural Machinery 2014; 45(10): 53–59. doi: 10.6041/j.issn.1000-1298.2014.10.009
3. Faiçal BS, Freitas H, Gomes PH, et al. An adaptive approach for UAV-based pesticide spraying in dynamic environments. Computers and Electronics in Agriculture 2017; 138: 210–223. doi: 10.1016/j.compag.2017.04.011
4. Li L, Liu Y, He X, et al. Assessment of spray deposition and losses in the apple orchard from agricultural unmanned aerial vehicle in China. 2018 ASABE Annual International Meeting 2018; 1800504. doi:10.13031/aim.201800504
5. Huang Y, Hoffmann WC, Lan Y, et al. Development of a spray system for an unmanned aerial vehicle platform. Applied Engineering in Agriculture 2009; 25(6): 803–809. doi: 10.13031/2013.29229
6. He Y, Wu J, Fang H, et al. Research on deposition effect of droplets based on plant protection unmanned aerial vehicle: A review. Journal of Zhejiang University (Agriculture and Life Sciences) 2018; 44(4): 392–398. doi: 10.3785/j.issn.1008-9209.2018.07.020
7. Liao J, Zang Y, Zhou Z, et al. Quality evaluation method and optimization of operating parameters in crop aerial spraying technology. Transactions of the Chinese Society of Agricultural Engineering (Transactions of the CSAE) 2015; 31(Supp.2): 38–46.
8. Tang Q, Zhang R, Chen L, et al. Droplets movement and deposition of an eight–rotor agricultural UAV in downwash flow field. International Journal of Agricultural and Biological Engineering 2017; 10(3): 47–56. doi: 10.3965/j.ijabe.20171003.3075
9. Zhou Q, Xue X, Qin W, et al. Optimization and test for structural parameters of UAV spraying rotary cup atomizer. International Journal of Agricultural and Biological Engineering 2017; 10(3): 78–86. doi: 10.3965/j.ijabe.20171003.3119
10. Zhang R, Zhang Z, Xu G, et al. Effect of spray adjuvant types and concentration on nozzle atomization. Transactions of the Chinese Society of Agricultural Engineering (Transactions of the CSAE) 2018; 34(20): 36–43.
11. Zhang J, He X, Song J, et al. Influence of spraying parameters of unmanned aircraft on droplets deposition. Transactions of the Chinese Society for Agricultural Machinery 2012; 43(12): 94–96. doi: 10.6041/j.issn.1000-1298.2012.12.017
12. Li J, Lan Y, Shi Y. Research progress on airflow characteristics and field pesticide application system of rotary-wing UAV. Transactions of the Chinese Society of Agricultural Engineering (Transactions of the CSAE) 2018; 34(12): 104–118. doi: 10.11975/j.issn.1002-6819.2018.12.013
13. Zhang P, Li Q, Yi S L, et al. Evaluation of spraying effect using small unmanned aerial vehicle (UAV) in citrus orchard. Journal of Fruit Science 2016; 33(1): 34–42.
14. Yang Z, Ge L, Qi L, et al. Influence of UAV rotor down-wash airflow on spray width. Transactions of the Chinese Society for Agricultural Machinery 2018; 49(1): 116–122. doi: 10.6041/j.issn.1000-1298.2018.01.014
15. Wen S, Han J, Lan Y, et al. Influence of wing tip vortex on drift of single rotor plant protection unmanned aerial vehicle. Transactions of the Chinese Society for Agricultural Machinery 2018; 49(8): 127–137. doi: 10.6041/j.issn.1000-1298.2018.08.015
16. Nuyttens D, Taylor WA, De Schampheleire M, et al. Influence of nozzle type and size on drift potential by means of different wind tunnel evaluation methods. Biosystems Engineering 2009; 103(3): 271–280. doi: 10.1016/j.biosystemseng.2009.04.001
17. Ru Y, Zhu C, Bao R. Spray drift model of droplets and analysis of influencing factors based on wind tunnel. Transactions of the Chinese Society for Agricultural Machinery 2014; 45(10): 66–72. doi: 10.6041/j.issn.1000-1298.2014.10.011
18. Zhang R, Li L, Fu W, et al. Spraying atomization performance by pulse width modulated variable and droplet deposition characteristics in wind tunnel. Transactions of the Chinese Society of Agricultural Engineering (Transactions of the CSAE) 2019; 35(3): 42–51. doi: 10.11975/j.issn.1002-6819.2019.03.006
19. Delele MA, Jaeken P, Debaer C, et al. CFD prototyping of an air-assisted orchard sprayer aimed at drift reduction. Computers and Electronics in Agriculture 2007; 55(1): 16–27. doi: 10.1016/j.compag.2006.11.002
20. Zheng Y, Yang S, Zhao C, et al. Modelling operation parameters of UAV on spray effects at different growth stages of corns. International Journal of Agricultural and Biological Engineering 2017; 10(3): 57–66. doi: 10.3965/j.ijabe.20171003.2578
21. Duga AT, Delele MA, Ruysen K, et al. Development and validation of a 3D CFD model of drift and its application to air-assisted orchard sprayers. Biosystems Engineering 2017; 154: 62–75. doi: 10.1016/j.biosystemseng.2016.10.010
22. Zhang B, Tang Q, Chen L ping, et al. Numerical simulation of spray drift and deposition from a crop spraying aircraft using a CFD approach. Biosystems Engineering 2018; 166: 184–199. doi: 10.1016/j.biosystemseng.2017.11.017
23. Qiu B, Wang L, Cai D, et al. Effects of flight altitude and speed of unmanned helicopter on spray deposition uniform. Transactions of the Chinese Society of Agricultural Engineering (Transactions of the CSAE) 2013; 29(24): 25–32. doi: 10.3969/j.issn.1002-6819.2013.24.004
24. Qin W, Xue X, Zhou L, et al. Effects of spraying parameters of unmanned aerial vehicle on droplets deposition distribution of maize canopies. Transactions of the Chinese Society of Agricultural Engineering (Transactions of the CSAE) 2014; 30(5): 50–56. doi: 10.3969/j.issn.1002-6819.2014.05.007
25. Chen S, Lan Y, Li J, et al. Effect of wind field below unmanned helicopter on droplet deposition distribution of aerial spraying. International Journal of Agricultural and Biological Engineering 2017; 10(3): 67–77. doi: 10.3965/j.ijabe.20171003.3078
26. Wang C, Song J, He X, et al. Effect of flight parameters on distribution characteristics of pesticide spraying droplets deposition of plant-protection unmanned aerial vehicle. Transactions of the Chinese Society of Agricultural Engineering (Transactions of the CSAE) 2017; 33(23): 109–116. doi: 10.11975/j.issn.1002-6819.2017.23.014
27. Torrent X, Garcerá C, Moltó E, et al. Comparison between standard and drift reducing nozzles for pesticide application in citrus: Part I. Effects on wind tunnel and field spray drift. Crop Protection 2017; 96: 130–143. doi: 10.1016/j.cropro.2017.02.001
28. Sharda A, Karkee M, Zhang Q, et al. Effect of emitter type and mounting configuration on spray coverage for solid set canopy delivery system. Computers and Electronics in Agriculture 2015; 112: 184–192. doi: 10.1016/j.compag.2014.07.012
29. Kesterson M, Luck J, Sama M. Development and preliminary evaluation of a spray deposition sensing system for improving pesticide application. Sensors 2015; 15(12): 31965–31972. doi: 10.3390/s151229898
30. Bae Y, Koo YM. Flight attitudes and spray patterns of a roll-balanced agricultural unmanned helicopter. Applied Engineering in Agriculture 2013; 29(5): 675–682. doi: 10.13031/aea.29.10059
31. Zhang R, Wen Y, Yi T, et al. Development and application of aerial spray droplets deposition performance measurement system based on spectral analysis technology. Transactions of the Chinese Society of Agricultural Engineering (Transactions of the CSAE) 2017; 33(24): 80–87. doi: 10.11975/j.issn.1002-6819.2017.24.011
32. Wen Y, Zhang R, Chen L, et al. A new spray deposition pattern measurement system based on spectral analysis of a fluorescent tracer. Computers and Electronics in Agriculture 2019; 160: 14–22. doi: 10.1016/j.compag.2019.03.008
33. Seddon J, Newman S. Basic Helicopter Aerodynamics, 3rd ed. Aerodynamics & Flight Mechanics Group; 2011. doi: 10.2514/4.868610
34. Hjelmfelt AT, Mockros LF. Motion of discrete particles in a turbulent fluid. Applied Scientific Research 1966; 16(1): 149–161. doi: 10.1007/bf00384062
35. Giles D, Billing R. Deployment and performance of a UAV for crop spraying. Chemical Engineering Transactions 2015; 44: 307–312. doi: 10.3303/CET1544052
36. Qin WC, Qiu BJ, Xue XY, et al. Droplet deposition and control effect of insecticides sprayed with an unmanned aerial vehicle against plant hoppers. Crop Protection 2016; 85: 79–88. doi: 10.1016/j.cropro.2016.03.018
37. Wang J, Luo B, Huo Y, et al. Influence of adjuvant on large-scale plant protection UAV spray characteristics. Journal of Drainage and Irrigation Machinery Engineering 2019; 37(12): 1044–1049.
38. Tang Y, Hou CJ, Luo SM, et al. Effects of operation height and tree shape on droplet deposition in citrus trees using an unmanned aerial vehicle. Computers and Electronics in Agriculture 2018; 148: 1–7. doi: 10.1016/j.compag.2018.02.026
39. He X. Brief analysis on the research, development and application of plant protection UAV in China. Pesticide Science and Administration 2018; 39(9): 20–27.
40. He X. Spray system and application technology of plant protection UAV in China. Agricultural Engineering Technology 2018; 38(9): 33–38. doi:10.16815/j.cnki.11-5436/s.2018.09.006
41. Xu G, Chen L, Zhang R. An image processing system for evaluation of aerial application quality. In: Proceedings of the 2016 International Conference on Intelligent Information Processing; 23–25 December 2016; Wuhan, China.
Refbacks
- There are currently no refbacks.
Copyright (c) 2021 Ruirui Zhang, Longlong Li, Yao Wen, Liping Chen, Qing Tang, Tongchuan Yi, Jiaxing Song

This work is licensed under a Creative Commons Attribution 4.0 International License.
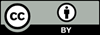
This site is licensed under a Creative Commons Attribution 4.0 International License (CC BY 4.0).
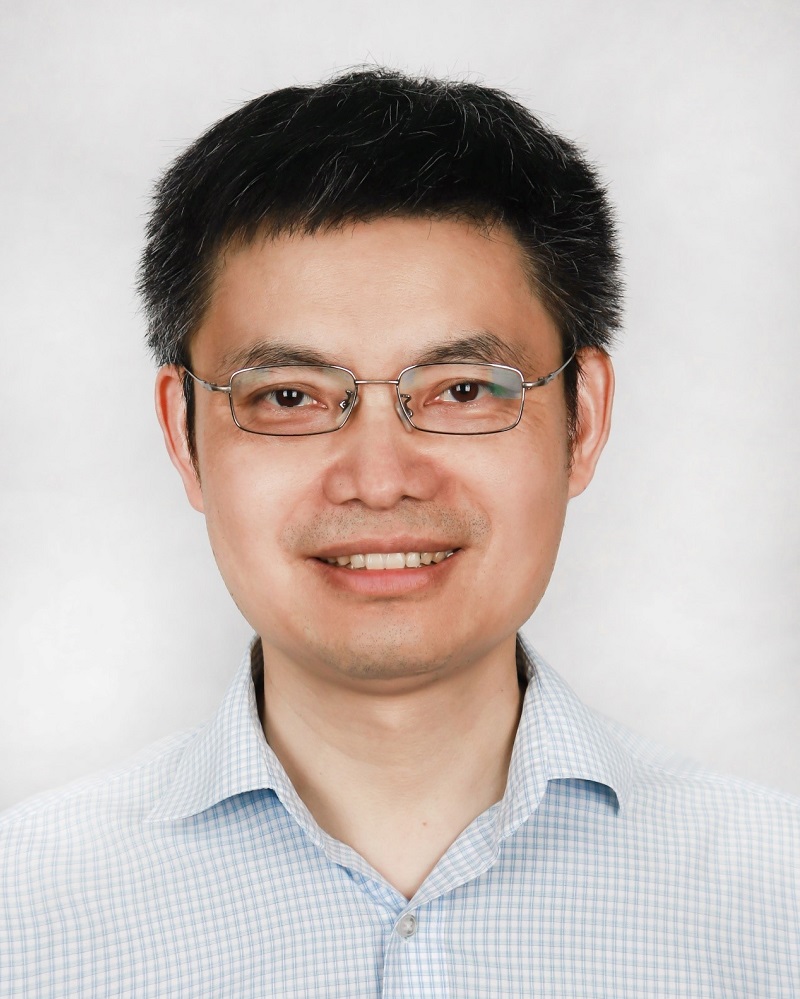
Prof. Zhengjun Qiu
Zhejiang University, China
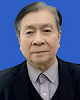
Cheng Sun
Academician of World Academy of Productivity Science; Executive Chairman, World Confederation of Productivity Science China Chapter, China
Processing Speed
-
-
-
- <5 days from submission to initial review decision;
- 64% acceptance rate
-
-
Modern agricultural technology is evolving rapidly, with scientists collaborating with leading agricultural enterprises to develop intelligent management practices. These practices utilize advanced systems that provide tailored fertilization and treatment options for large-scale land management.
This journal values human initiative and intelligence, and the employment of AI technologies to write papers that replace the human mind is expressly prohibited. When there is a suspicious submission that uses AI tools to quickly piece together and generate research results, the editorial board of the journal will reject the article, and all journals under the publisher's umbrella will prohibit all authors from submitting their articles.
Readers and authors are asked to exercise caution and strictly adhere to the journal's policy regarding the usage of Artificial Intelligence Generated Content (AIGC) tools.
Asia Pacific Academy of Science Pte. Ltd. (APACSCI) specializes in international journal publishing. APACSCI adopts the open access publishing model and provides an important communication bridge for academic groups whose interest fields include engineering, technology, medicine, computer, mathematics, agriculture and forestry, and environment.