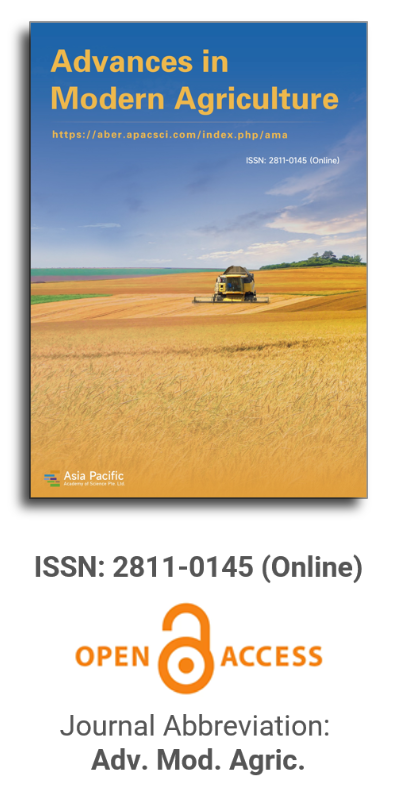
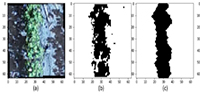
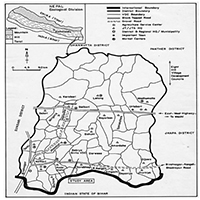
Rhizospheric bacteria with potential benefits in agriculture
Vol 3, Issue 1, 2022
VIEWS - 2015 (Abstract)
Download PDF
Abstract
Rhizobacteria are a vast and very diverse group of bacteria that live in the vicinity of roots. They develop beneficial, neutral, and even detrimental relationships, although the latter to a lesser extent. The interactions between bacteria and plant roots have played a determining role in the adaptation and productivity of plant species over time. Several studies show that rhizobacteria have improved plant growth, production, and health, both directly through mechanisms that include the assimilation of vital nutrients such as nitrogen fixation, phosphorus and potassium solubilization, and phytostimulation through the production of various phytohormones) and indirectly (by affecting the growth of important pathogens, activating plant immunity, and improving problems caused by abiotic stress). Due to their metabolic diversity, rhizobacteria could contribute positively to the improvement of agricultural productivity and the solution of environmental problems caused by the methods used in current agriculture. Several genera, such as Acidithiobacillus, Aminobacter, Arthrobacter, Azoarcus, Azospirillum, Azotobacter, Bacillus, Burkholderia, Clostridium, Enterobacter, Gluconoacetobacter, Pseudomonas, Serratia, and Sphingomonas, have demonstrated their enormous growth-promoting capacity. This review provides a focus on the mechanisms by which rhizobacteria enhance plant growth, their contribution to sustainable agriculture, and their commercialization, a field that continues to grow steadily.
Keywords
References
1. Food and Agriculture Organization of the United Nations. The future of food and agriculture: Trends and challenges. Available online: http://www.fao.org/3/a- i6881s.pdf (accessed on 3 March 2019).
2. Shailendra Singh GG. Plant growth promoting rhizobacteria (PGPR): Current and future prospects for development of sustainable agriculture. Journal of Microbial & Biochemical Technology 2015; 7(2). doi: 10.4172/1948-5948.1000188
3. Pretty J, Benton TG, Bharucha ZP, et al. Global assessment of agricultural system redesign for sustainable intensification. Nature Sustainability 2018; 1(8): 441–446. doi: 10.1038/s41893-018-0114-0
4. Werner GDA, Cornwell WK, Sprent JI, et al. A single evolutionary innovation drives the deep evolution of symbiotic N2-fixation in angiosperms. Nature Communications 2014; 5(1). doi: 10.1038/ncomms5087
5. Upson JL, Zess EK, Bialas A, et al. The coming of age of EvoMPMI: Evolutionary molecular plant-microbe interactions across multiple timescales. Current Opinion in Plant Biology 2018; 44: 108–116. doi: 10.1016/j. pbi.2018.03.003
6. Stringlis IA, Proietti S, Hickman R, et al. Root transcriptional dynamics induced by beneficial rhizobacteria and microbial immune elicitors reveal signatures of adaptation to mutualists. The Plant Journal 2017; 93(1): 166–180. doi: 10.1111/tpj.13741
7. Molina-Romero D, Bustillos-Cristales MR, Rodríguez-Andrade O, et al. Mechanisms of phytostimulation by rhizobacteria, isolates in America and biotechnological potential (Spanish). Biologicas 2015; 17(2): 24–34.
8. McNear DH. The rhizosphere - roots, soil and everything in between. Available online: https://www.researchgate.net/publication/303919396_The_rhizosphere-roots_soil_and_everything_in_between (accessed on 19 December 2019).
9. Kloepper JW, Schroth MN. Plant growth-promoting rhizobacteria on radishes. In: Proceedings of the 4th International Conference on Plant Pathogenic Bacteria; 27 August–2 September 1978; Angers, France. pp. 879–882.
10. Venturi V, Keel C. Signaling in the rhizosphere. Trends Plant Science 2016; 21: 187–198. doi: 10.1016/j. tplants.2016.01.005
11. Ahemad M, Kibret M. Mechanisms and applications of plant growth promoting rhizobacteria: Current perspective. Journal of King Saud University – Science 2014; 26(1): 1–20. doi: 10.1016Zj. jksus.2013.05.001
12. Mayz J. Biological nitrogen fixation. Revista Cientifica UDO Agricola 2004; 4: 1–20.
13. Bhattacharyya PN, Jha DK. Plant growth-promoting rhizobacteria (PGPR): Emergence in agriculture. World Journal of Microbiology and Biotechnology 2011; 28(4): 1327–1350. doi: 10.1007/s11274-011-0979-9
14. Perin L, Martínez-Aguilar L, Castro-González R, et al. Diazotrophic Burkholderia species associated with field-grown maize and sugarcane. Applied and Environmental Microbiology 2006; 72: 3103–3110. doi: 10.1128/ aem.72.5.3103-3110.2006
15. Razaq M, Zhang P, Shen H long, et al. Influence of nitrogen and phosphorous on the growth and root morphology of Acer mono. PLoS ONE 2017; 12(2): e0171321. doi: 10.1371/journal.pone.0171321
16. Weil RR, Brady NC. The Nature and Properties of Soils. Prentice Hall; 2002.
17. Yadav BK, Verm A. Phosphate solubilization and mobilization in soil through microorganisms under arid ecosystems. In: Ali M (editor). The Functioning of Ecosystems. IntechOpen; 2012. doi: 10.5772/35917
18. Lugtenberg B, Kamilova F. Plant-growth-promoting rhizobacteria. Annual Review of Microbiology 2009; 63(1): 541–556. doi: 10.1146/annurev.micro.62.081307.162918
19. Oteino N, Lally RD, Kiwanuka S, et al. Plant growth promotion induced by phosphate solubilizing endophytic Pseudomonas isolates. Frontiers in Microbiology 2015; 6: 1–9. doi: 10.3389/ fmicb.2015.00745
20. Khan MS, Zaidi A, Wani PA. Role of phosphate-solubilizing microorganisms in sustainable agriculture—A review. Agronomy for Sustainable Development 2007; 27: 29–43. doi: 10.1051/agro:2006011
21. Parmar P, Sindhu SS. Potassium solubilization by rhizosphere bacteria: Influence of nutritional and environmental conditions. Journal of Microbiology Research 2013; 3: 25–31. doi: 10.5923/j.microbiology.20130301.04
22. Gouda S, Kerry RG, Das G, et al. Revitalization of plant growth promoting rhizobacteria for sustainable development in agriculture. Microbiological Research 2018; 206: 131–140. doi: 10.1016/j. micres.2017.08.016
23. Ahmad MS, Zargar MY. Characterization of potassium solubilizing bacteria (KSB) in rhizospheric soils of apple (Malus domestica Borkh.) in temperate Kashmir. Journal of Applied Life Sciences International 2017; 15: 1–7. doi: 10.9734/ JALSI/2017/36848
24. Bagyalakshmi B, Ponmurugan P, Balamurugan A. Potassium solubilization, plant growth promoting substances by potassium solubilizing bacteria (KSB) from southern Indian tea plantation soil. Biocatalysis and Agricultural Biotechnology 2017; 12: 116–124. doi: 10.1016/j.bcab.2017.09.011
25. Bakhshandeh E, Rahimian H, Pirdashti H, et al. Phosphate solubilization potential and modeling of stress tolerance of rhizobacteria from rice paddy soil in northern Iran. World Journal of Microbiology and Biotechnology 2014; 30(9): 2437–2447. doi: 10.1007/s11274-014-1669-1
26. Abou-el-Seoud II, Abdel-Megeed A. Impact of rock materials and biofertilizations on P and K availability for maize (Zea Maize) under calcareous soil conditions. Saudi Journal of Biological Sciences 2012; 19(1): 55–63. doi: 10.1016/j.sjbs.2011.09.001
27. Zhang C, Kong F. Isolation and identification of potassium-solubilizing bacteria from tobacco rhizospheric soil and their effect on tobacco plants. Applied Soil Ecology 2014; 82: 18–25. doi: 10.1016/j.apsoil.2014.05.002
28. Singh G, Biswas DR, Marwaha TS. Mobilization of potassium from waste mica by plant growth promoting rhizobacteria and its assimilation by maize (Zea mays) and wheat (Triticum aestivum L.): A hydroponics study under phytotron growth chamber. Journal of Plant Nutrition 2010; 33(8): 1236–1251. doi: 10.1080/01904161003765760
29. Saiyad SA, Jhala YK, Vyas RV. Comparative efficiency of five potash and phosphate solubilizing bacteria and their key enzymes useful for enhancing and improvement of soil fertility. International Journal of Scientific and Research Publications 2015; 5(2): 1–6.
30. Etesami H, Emami S, Alikhani HA. Potassium solubilizing bacteria (KSB): Mechanisms, promotion of plant growth, and future prospects—A review. Journal of Soil Science and Plant Nutrition 2017; 17(4): 897–911. doi: 10.4067/S0718- 95162017000400005
31. Damam M, Kaloori K, Gaddam B, Kausar R. Plant growth promoting substances (phytohormones) produced by rhizobacterial strains isolated from the rhizosphere of medicinal plants. International Journal of Pharmaceutical Sciences Review and Research 2016; 37(1): 130–136.
32. Calvo P, Ormeño-Orrillo E, Martínez-Romero E, et al. Characterization of Bacillus isolates of potato rhizosphere from andean soils of Peru and their potential PGPR characteristics. Brazilian Journal of Microbiology 2010; 41(4): 899–906. doi: 10.1590/s1517-83822010000400008
33. Carcaño-Montiel MG, Ferrera-Cerrato R, Pérez-Moreno J, et al. Nitrogenase activity, production of phytohormones, siderophores and antibiosis in strains of Azospirillum and Klebsiella isolated from maize and teosintle. Terra Latinoamericana 2006; 24(4): 493–502.
34. Angulo VC, Sanfuentes EA, Rodríguez F, Sossa KE. Characterization of growth-promoting rhizobacteria in Eucalyptus nitens seedlings (Spanish). Revista Argentina de Microbiología 2014; 46: 338–347. doi: 10.1016/S0325-7541(14)70093-8
35. Pereira SIA, Monteiro C, Vega AL, et al. Endophytic culturable bacteria colonizing Lavandula dentata L. plants: Isolation, characterization and evaluation of their plant growth-promoting activities. Ecological Engineering 2016; 87: 91–97. doi: 10.1016/j.ecoleng.2015.11.033
36. Egamberdieva D, Wirth SJ, Alqarawi AA, et al. Phytohormones and beneficial microbes: Essential components for plants to balance stress and fitness. Frontiers in Microbiology 2017; 8: 2104. doi: 10.3389/ fmicb.2017.02104
37. Fibach-Paldi S, Burdman S, Okon Y. Key physiological properties contributing to rhizosphere adaptation and plant growth promotion abilities of Azospirillum brasilense. FEMS Microbiology Letters 2012; 326(2): 99–108. doi: 10.1111/ j.1574-6968.2011.02407.x. c
38. Asgher Mohd, Khan MIR, Anjum NA, et al. Minimising toxicity of cadmium in plants—Role of plant growth regulators. Protoplasma 2014; 252(2): 399–413. doi: 10.1007/s00709-014-0710-4
39. Ali B. Bacterial auxin signaling: Comparative study of growth induction in Arabidopsis thaliana and Triticum aestivum. Turkish Journal of Botany 2015; 39(1): 1–9. doi. 10.3906/bot- 1401-31
40. Raheem A, Shaposhnikov A, Belimov A, et al. Auxin production by rhizobacteria was associated with improved yield of wheat (Triticum aestivum L.) under drought stress. Archives of Agronomy and Soil Science 2018; 64(4): 574–587. doi: 10.1 080/03650340.2017.1362105
41. Kang SM, Waqas M, Hamayun M, et al. Gibberellins and indole-3-acetic acid producing rhizospheric bacterium Leifsonia xyli SE134 mitigates the adverse effects of copper-mediated stress on tomato. Journal of Plant Interactions 2017; 12(1): 373–380. doi: 10.1080/17429145.2017.1370142
42. Liu F, Xing S, Ma H, et al. Cytokinin-producing, plant growth-promoting rhizobacteria that confer resistance to drought stress in Platycladus orientalis container seedlings. Applied Microbiology and Biotechnology 2013; 97(20): 9155–9164. doi: 10.1007/s00253-013-5193-2
43. Phillips DA, Torrey JG. Studies on cytokinin production by rhizobium. Plant Physiology 1972; 49(1): 11–15. doi: 10.1104/pp.49.1.11
44. Vacheron J, Desbrosses G, Bouffaud ML, et al. Plant growth-promoting rhizobacteria and root system functioning. Frontiers in Plant Science 2013; 4. doi: 10.3389/fpls.2013.00356
45. Maheshwari DK, Dheeman S, Agarwal M. Phytohormone-producing PGPR for sustainable agriculture. In: Maheshwari DK (editor). Bacterial Metabolites in Sustainable Agroecosystem. Springer; 2015. Volume 12. pp. 159–182. doi: 10.1007/978- 3-319-24654-3-3_7
46. Ahmad P. Growth and antioxidant responses in mustard (Brassica juncea L.) plants subjected to combined effect of gibberellic acid and salinity. Archives of Agronomy and Soil Science 2010; 56(5): 575–588. doi: 10.1080/03650340903164231
47. Iqbal M, Ashraf M. Gibberellic acid mediated induction of salt tolerance in wheat plants: Growth, ionic partitioning, photosynthesis, yield and hormonal homeostasis. Environmental and Experimental Botany 2013; 86: 76–85. doi: 10.1016/j. envexpbot.2010.06.002
48. Desai SA. Isolation and characterization of gibberellic acid (GA3) producing rhizobacteria from sugarcane roots. Bioscience Discovery 2017; 8(3): 488–494.
49. Cohen AC, Bottini R, Piccoli P. Role of abscisic acid producing PGPR in sustainable agriculture. In: Maheshwari DK (editor). Bacterial Metabolites in Sustainable Agroecosystem. Springer; 2015. Volume 12. pp. 259–282. doi: 10.1007/978-3-319- 24654-3_9
50. Zhou C, Li F, Xie Y, et al. Involvement of abscisic acid in microbe-induced saline-alkaline resistance in plants. Plant Signaling & Behavior 2017; 12(10): e1367465. doi: 10.1080/15592324.2017.1367465
51. Sah SK, Reddy KR, Li J. Abscisic acid and abiotic stress tolerance in crop plants. Frontiers in Plant Science 2016; 7. doi: 10.3389/fpls.2016.00571
52. Shahzad R, Khan AL, Bilal S, et al. Inoculation of abscisic acid-producing endophytic bacteria enhances salinity stress tolerance in Oryza sativa. Environmental and Experimental Botany 2017; 136: 68–77. doi: 10.1016/j. envexpbot.2017.01.010
53. Li X, Cai J, Liu F, et al. Exogenous abscisic acid application during grain filling in winter wheat improves cold tolerance of offspring’s seedlings. Journal of Agronomy and Crop Science 2014; 200(6): 467–478. doi: 10.1111/jac.12064
54. Mohammed AE. Effectiveness of exopolysaccharides and biofilm forming plant growth promoting rhizobacteria on salinity tolerance of faba bean (Vicia faba L.). African Journal of Microbiology Research 2018; 12(17): 399–404. doi: https://doi.org/10.5897/ AJMR2018.8822
55. Upadhyay SK, Singh JS, Singh DP. Exopolysaccharide-producing plant growth-promoting rhizobacteria under salinity condition. Pedosphere 2011; 21(2): 214–222. doi: 10.1016/s1002-0160(11)60120-3
56. Gupta G, Snehi SK, Singh V. Role of PGPR in biofilm formations and its importance in plant health. In: Gupta G, Snehi SK, Singh V (editors). Biofilms in Plant and Soil Health. John Wiley & Sons; 2017. pp. 27–42. doi: 10.1002/9781119246329.ch2
57. Kasim WA, Gaafar RM, Abou-Ali RM, et al. Effect of biofilm forming plant growth promoting rhizobacteria on salinity tolerance in barley. Annals of Agricultural Sciences 2016; 61: 217–227. doi: 10.1016/j. aoas.2016.07.003
58. Mahmood S, Daur I, Al-Solaimani SG, et al. Plant growth promoting rhizobacteria and silicon synergistically enhance salinity tolerance of mung bean. Frontiers in Plant Science 2016; 7. doi: 10.3389/fpls.2016.00876
59. Naseem H, Ahsan M, Shahid MA, et al. Exopolysaccharides producing rhizobacteria and their role in plant growth and drought tolerance. Journal of Basic Microbiology 2018; 58(12): 1009–1022. doi: 10.1002/jobm.201800309
60. Foyer CH, Rasool B, Davey JW, et al. Cross-tolerance to biotic and abiotic stresses in plants: A focus on resistance to aphid infestation. Journal of Experimental Botany 2016; 67(7): 2025–2037. doi: 10.1093/jxb/erw079
61. Barnawal D, Pandey SS, Bharti N, et al. ACC deaminase-containing plant growth-promoting rhizobacteria protect papaver somniferum from downy mildew. Journal of Applied Microbiology 2017; 122(5): 1286–1298. doi: 10.1111/jam.13417
62. Stearns JC, Woody OZ, McConkey BJ, et al. Effects of bacterial ACC deaminase on brassica napus gene expression. Molecular Plant-Microbe Interactions® 2012; 25(5): 668–676. doi: 10.1094/mpmi-08-11-0213
63. Sarkar A, Ghosh PK, Pramanik K, et al. A halotolerant Enterobacter sp. displaying ACC deaminase activity promotes rice seedling growth under salt stress. Research in Microbiology 2018; 169(1): 20–32. doi: 10.1016/j.resmic.2017.08.005
64. Shen M, Jun Kang Y, Li Wang H, et al. Effect of plant growth-promoting rhizobacteria (PGPRs) on plant growth, yield, and quality of tomato (Lycopersicon esculentum Mill.) under simulated seawater irrigation. The Journal of General and Applied Microbiology 2012; 58(4): 253–262. doi: 10.2323/jgam.58.253
65. Cedeño-García GA, Gerding M, Moraga G, et al. Plant growth promoting rhizobacteria with ACC deaminase activity isolated from Mediterranean dryland areas in Chile: Effects on early nodulation in alfalfa. Chilean Journal of Agricultural Research 2018; 78(3): 360–369. doi: 10.4067/s0718-58392018000300360
66. Patil C, Suryawanshi R, Koli S, et al. Improved method for effective screening of ACC (1-aminocyclopropane-1-carboxylate) deaminase producing microorganisms. Journal of Microbiological Methods 2016; 131: 102–104. doi: 10.1016/j.mimet.2016.10.009
67. Vurukonda SSKP, Vardharajula S, Shrivastava M, et al. Enhancement of drought stress tolerance in crops by plant growth promoting rhizobacteria. Microbiological Research 2016; 184: 13–24. doi: 10.1016/j.micres.2015.12.003
68. Ali SZ, Sandhya V, Grover M, et al. Effect of inoculation with a thermotolerant plant growth promoting Pseudomonas putida strain AKMP7 on growth of wheat (Triticum spp.) under heat stress. Journal of Plant Interactions 2011; 6(4): 239–246. doi: 10.1080/17429145.2010.545147
69. Palacio-Rodríguez R, Ramos BP, Coria-Arellano JL, et al. Mechanisms of PGPRs to mitigate plant abiotic stress. Arid-Science 2016; 1: 4–11.
70. Grover M, Ali SKZ, Sandhya V, et al. Role of microorganisms in adaptation of agriculture crops to abiotic stresses. World Journal of Microbiology and Biotechnology 2010; 27(5): 1231–1240. doi: 10.1007/s11274-010-0572-7
71. Lucas JA, García-Villaraco A, Ramos B, et al. Structural and functional study in the rhizosphere of Oryza sativa L. plants growing under biotic and abiotic stress. Journal of Applied Microbiology 2013; 115(1): 218–235. doi: 10.1111/jam.12225
72. Chaturvedi S. Induced systemic resistance. Open Access Journal of Microbiology & Biotechnology 2018; 3(1). doi: 10.23880/oajmb-16000126
73. Sunar K, Dey P, Chakraborty U, Chakraborty B. Biocontrol efficacy and plant growth promoting activity of Bacillus altitudinis isolated from Darjeeling hills. Journal of Basic Microbiology 2013; 55(1): 91–104. doi: 10.1002/ jobm.201300227
74. Poupin MJ, Timmermann T, Vega A, et al. Effects of the plant growth-promoting bacterium Burkholderia phytofirmans PsJN throughout the life cycle of Arabidopsis thaliana. PLoS ONE 2013; 8(7): e69435. doi: 10.1371/journal.pone.0069435
75. Bloemberg GV, Lugtenberg BJ. Molecular basis of plant growth promotion and biocontrol by rhizobacteria. Current Opinion in Plant Biology 2001; 4(4): 343–350. doi: 10.1016/S1369-5266(00)00183-7
76. Saleem M, Asghar HN, Zahir ZA, et al. Impact of lead tolerant plant growth promoting rhizobacteria on growth, physiology, antioxidant activities, yield and lead content in sunflower in lead contaminated soil. Chemosphere 2018; 195: 606–614. doi: 10.1016/j.chemosphere.2017.12.117
77. Kim JS, Lee JE, Nie H, et al. Physiological and proteomic analysis of plant growth enhancement by the rhizobacterium Bacillus sp. JS. Genes & Genomics 2018; 40: 129–136. doi: 10.1007/s13258- 017-0615-7
78. Kuan KB, Othman R, Abdul Rahim K, Shamsuddin ZH. Plant growth-promoting rhizobacteria inoculation to enhance vegetative growth, nitrogen fixation and nitrogen remobilization of maize under greenhouse conditions. PLoS ONE 2016; 11: 1–19. doi: 10.1371/journal. pone.0152478
79. Morgado González A, Espinosa Victoria D, Gómez Merino FC. Efficiency of plant growth promoting rhizobacteria (PGPR) in sugarcane. Terra Latinoamericana 2015; 33(4): 321–330.
80. Jahanian A, Chaichi MR, Rezaei K, et al. The effect of plant growth promoting rhizobacteria (PGPR) on germination and primary growth of artichoke (Cynara scolymus). International Journal of Agriculture and Crop Sciences 2012; 4: 923–929.
81. Sharma SK. Selection of plant growth-promoting Pseudomonas spp. That enhanced productivity of soybean-wheat cropping system in central India. Journal of Microbiology and Biotechnology 2011; 21(11): 1127–1142. doi: 10.4014/jmb.1012.12018
82. Dary M, Chamber-Pérez MA, Palomares AJ, et al. “In situ” phytostabilisation of heavy metal polluted soils using Lupinus luteus inoculated with metal resistant plant-growth promoting rhizobacteria. Journal of Hazardous Materials 2010; 177(1–3): 323–330. doi: 10.1016/j.jhazmat.2009.12.035
83. Gholami A, Shahsavani S, Nezarat S. The effect of plant growth promoting rhizobacteria (PGPR) on germination, seedling growth and yield of maize. International Journal of Agricultural and Biosystems Engineering 2009; 3(1): 35–40.
84. Rodrigues EP, Rodrigues LS, de Oliveira ALM, et al. Azospirillum amazonense inoculation: Effects on growth, yield and N2 fixation of rice (Oryza sativa L.). Plant and Soil 2007; 302(1–2): 249–261. doi: 10.1007/s11104-007-9476-1
85. Lawongsa P, Boonkerd N, Wongkaew S, et al. Molecular and phenotypic characterization of potential plant growth-promoting Pseudomonas from rice and maize rhizospheres. World Journal of Microbiology and Biotechnology 2008; 24(9): 1877–1884. doi: 10.1007/s11274-008-9685-7
86. Remans R, Beebe S, Blair M, et al. Physiological and genetic analysis of root responsiveness to auxin-producing plant growth-promoting bacteria in common bean (Phaseolus vulgaris L.). Plant and Soil 2007; 302(1–2): 149–161. doi: 10.1007/s11104-007-9462-7
87. Adesemoye AO, Obini M, Ugoji EO. Comparison of plant growth-promotion with Pseudomonas aeruginosa and Bacillus subtilis in three vegetables. Brazilian Journal of Microbiology 2008; 39(3): 423–426. doi: 10.1590/s1517-83822008000300003
88. Valverde A, Burgos A, Fiscella T, et al. Differential effects of coinoculations with Pseudomonas jessenii PS06 (a phosphate-solubilizing bacterium) and Mesorhizobium ciceri C-2/2 strains on the growth and seed yield of chickpea under greenhouse and field conditions. Plant Soil 2006; 287: 43–50. doi: https://doi.org/10.1007/ s11104-006-9057-8
89. Wu CH, Wood TK, Mulchandani A, et al. Engineering plant-microbe symbiosis for rhizoremediation of heavy metals. Applied and Environmental Microbiology 2006; 72(2): 1129–1134. doi: 10.1128/aem.72.2.1129-1134.2006
90. Beneduzi A, Ambrosini A, Passaglia LMP. Plant growth promoting rhizobacteria (PGPR): Their potential as antagonists and biocontrol agents. Genetics and Molecular Biology 2012; 35: 1044–1051. doi: 10.1590/s1415-47572012000600020
91. Chuks Kenneth O. Plant growth promoting rhizobacteria (PGPR): A bioprotectant bioinoculant for sustainable agrobiology. A review. International Journal of Advanced Research in Biological Sciences (IJARBS) 2017; 4(5): 123–142. doi: 10.22192/ijarbs.2017.04.05.014
92. Tenorio-Salgado S, Tinoco R, Vazquez-Duhalt R, et al. Identification of volatile compounds produced by the bacterium Burkholderia tropica that inhibit the growth of fungal pathogens. Bioengineered 2013; 4(4): 236–243. doi: 10.4161/bioe.23808
93. Rajesh Kan R, Prakash Vi SG. Molecular characterization of antagonistic streptomyces isolated from a mangrove swamp. Asian Journal of Biotechnology 2011; 3(3): 237–245. doi: 10.3923/ajbkr.2011.237.245
94. Rajkumar M, Ae N, Prasad MNV, et al. Potential of siderophore-producing bacteria for improving heavy metal phytoextraction. Trends in Biotechnology 2010; 28(3): 142–149. doi: 10.1016/j.tibtech.2009.12.002
95. Farag MA, Zhang H, Ryu CM. Dynamic chemical communication between plants and bacteria through airborne signals: Induced resistance by bacterial volatiles. Journal of Chemical Ecology 2013; 39: 1007–1018. doi: 10.1007/s10886-013- 0317-9
96. Tahir HAS, Gu Q, Wu H, et al. Plant growth promotion by volatile organic compounds produced by Bacillus subtilis SYST2. Frontiers in Microbiology 2017; 8: 1–11. doi: 10.3389/ fmicb.2017.00171
Refbacks
- There are currently no refbacks.
Copyright (c) 2020 Antonio Velasco-Jiménez, Osvaldo Castellanos-Hernández, Gustavo Acevedo-Hernández, Rayn Clarenc Aarland, Araceli Rodríguez-Sahagún

This work is licensed under a Creative Commons Attribution 4.0 International License.
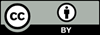
This site is licensed under a Creative Commons Attribution 4.0 International License (CC BY 4.0).
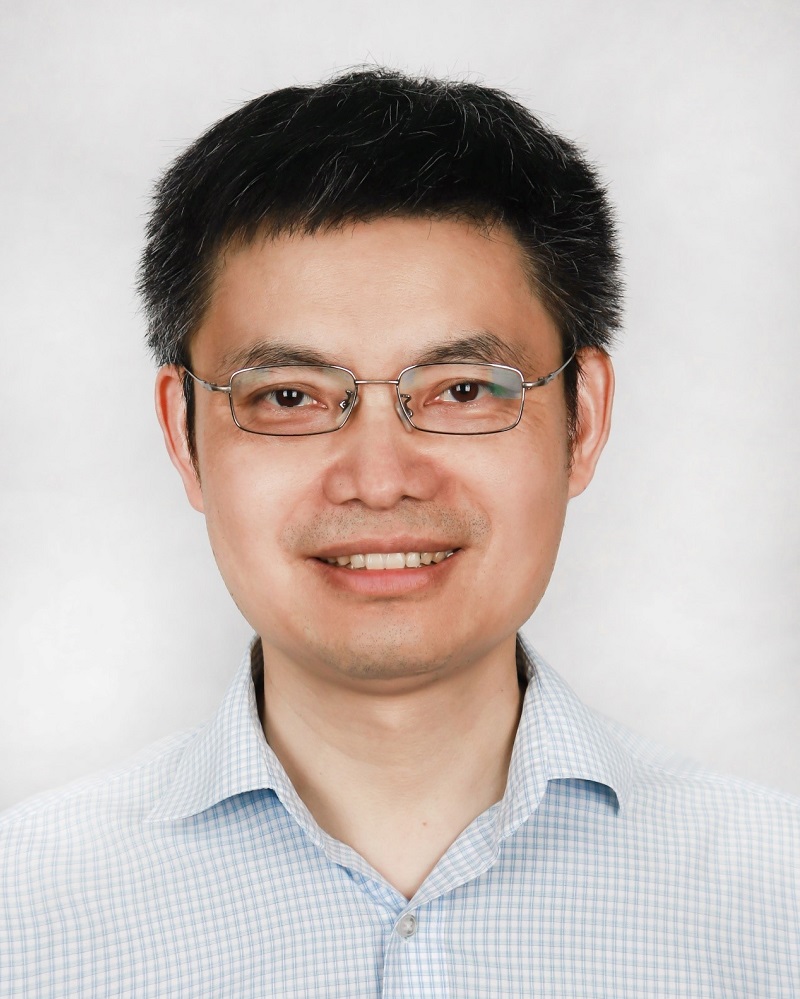
Prof. Zhengjun Qiu
Zhejiang University, China
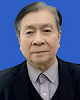
Cheng Sun
Academician of World Academy of Productivity Science; Executive Chairman, World Confederation of Productivity Science China Chapter, China
Processing Speed
-
-
-
- <5 days from submission to initial review decision;
- 64% acceptance rate
-
-
Modern agricultural technology is evolving rapidly, with scientists collaborating with leading agricultural enterprises to develop intelligent management practices. These practices utilize advanced systems that provide tailored fertilization and treatment options for large-scale land management.
This journal values human initiative and intelligence, and the employment of AI technologies to write papers that replace the human mind is expressly prohibited. When there is a suspicious submission that uses AI tools to quickly piece together and generate research results, the editorial board of the journal will reject the article, and all journals under the publisher's umbrella will prohibit all authors from submitting their articles.
Readers and authors are asked to exercise caution and strictly adhere to the journal's policy regarding the usage of Artificial Intelligence Generated Content (AIGC) tools.
Asia Pacific Academy of Science Pte. Ltd. (APACSCI) specializes in international journal publishing. APACSCI adopts the open access publishing model and provides an important communication bridge for academic groups whose interest fields include engineering, technology, medicine, computer, mathematics, agriculture and forestry, and environment.