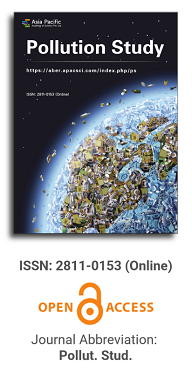
Asia Pacific Academy of Science Pte. Ltd. (APACSCI) specializes in international journal publishing. APACSCI adopts the open access publishing model and provides an important communication bridge for academic groups whose interest fields include engineering, technology, medicine, computer, mathematics, agriculture and forestry, and environment.
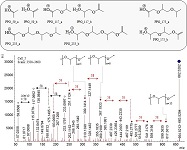
The (partial) replacement of synthetic polymers with bioplastics is due to increased production of conventional packaging plastics causing for severe environmental pollution with plastics waste. The bioplastics, however, represent complex mixtures of known and unknown (bio)polymers, fillers, plasticizers, stabilizers, flame retardant, pigments, antioxidants, hydrophobic polymers such as poly(lactic acid), polyethylene, polyesters, glycol, or poly(butylene succinate), and little is known of their chemical safety for both the environment and the human health. Polymerization reactions of bioplastics can produce no intentionally added chemicals to the bulk material, which could be toxic, as well. When polymers are used to food packing, then the latter chemicals could also migrate from the polymer to food. This fact compromises the safety for consumers, as well. The scarce data on chemical safety of bioplastics makes a gap in knowledge of their toxicity to humans and environment. Thus, development of exact analytical protocols for determining chemicals of bioplastics in environmental and food samples as well as packing polymers can only provide warrant for reliable conclusive evidence of their safety for both the human health and the environment. The task is compulsory according to legislation Directives valid to environmental protection, food control, and assessment of the risk to human health. The quantitative and structural determination of analytes is primary research task of analysis of polymers. The methods of mass spectrometry are fruitfully used for these purposes. Methodological development of exact analytical mass spectrometric tools for reliable structural analysis of bioplastics only guarantees their safety, efficacy, and quality to both humans and environment. This study, first, highlights innovative stochastic dynamics equations processing exactly mass spectrometric measurands and, thus, producing exact analyte quantification and 3D molecular and electronic structural analyses. There are determined synthetic polymers such as poly(ethylenglycol), poly(propylene glycol), and polyisoprene as well as biopolymers in bags for foodstuffs made from renewable cellulose and starch, and containing, in total within the 20,416–17,495 chemicals per sample of the composite biopolymers. Advantages of complementary employment in mass spectrometric methods and Fourier transform infrared spectroscopy is highlighted. The study utilizes ultra-high resolution electrospray ionization mass spectrometric and Fourier transform infrared spectroscopic data on biodegradable plastics bags for foodstuffs; high accuracy quantum chemical static methods, molecular dynamics; and chemometrics. There is achieved method performance |r| = 0.99981 determining poly(propylene glycol) in bag for foodstuff containing 20,416 species and using stochastic dynamics mass spectrometric formulas. The results highlight their great capability and applicability to the analytical science as well as relevance to both the fundamental research and to the industry.
Quarry operations and soil health: Tracing metal pollution in Awi and Njagachan communities, Nigeria
Vol 6, Issue 1, 2025
Download PDF
Abstract
This study investigated the influence of quarry operations on soil health by evaluating the metal pollution status of soils around major quarry communities (Awi and Njagachan) in Akamkpa, Nigeria. Soil samples were subjected to wet digestion and quantified using an Atomic Absorption Spectrophotometer (Model AA-6800, Japan). The concentrations of lead (63.34–96.34 mg/kg), cadmium (4.29–7.40 mg/kg), mercury (2.34–3.76 mg/kg), arsenic (2.48–5.21 mg/kg), and selenium (1.22–2.75 mg/kg) were all below the U.S. Environmental Protection Agency (US-EPA), Dutch remediation levels, and other soil guidelines. However, significant spatial variation in metal levels across quarry sites points to anthropogenic influences, with quarries likely contributing to the elevated metal concentrations. Contamination factors indicated moderate contamination by lead, considerable contamination by cadmium, and very high contamination by mercury. The degree of contamination was high for all quarries except Ding Zing quarry, which showed a very high degree during the wet season. Ecological risk assessment revealed low potential risk from lead and arsenic, moderate to high risk from cadmium, and very high risk from mercury. Geo-accumulation indices suggested that soils were largely unpolluted by lead and arsenic but ranged from unpolluted to moderately polluted by cadmium and mercury. The study concludes that quarry activities contribute to elevated metal concentrations, posing varying levels of ecological risk. Continuous monitoring is strongly recommended to prevent potential long-term human and environmental health risks, with a focus on addressing mercury contamination. Regulatory measures should be enforced to mitigate further pollution.
Keywords
References
- Ogbiji JE, Ogbiji A. Quarry industries and socio-economic development in some selected communities in Cross River State of Nigeria. World Wide Journal of Multidisciplinary Research & Development. 2016; 2(12): 10–14.
- Inyaka GI, Alkali M, Mba EH, et al. Assessment of dust concentration & compounds at quarry sites in Akamkpa Cross River State, Nigeria. Asian Journal of Geological Research. 2021; 4(1): 40–47.
- Akinola MO, Aiyesanmi AF. Environmental implications of rock quarrying activities in Nigeria. Journal of Environmental Science and Technology. 2015; 8(2): 45–56.
- Swain CK. Environmental pollution indices: a review on concentration of heavy metals in air, water, and soil near industrialization and urbanisation. Discover Environment. 2024; 2(5).
- Tiimub BM, Sarkodie PA, Monney A, et al. Heavy Metal Contamination of Soil by Quarry Dust at Asonomaso in the Ashanti Region of Ghana. Chemistry and Materials Research. 2015; 7: 42–50.
- Adewole AT, Adesina AG. Impact of Quarrying on Soil Quality in Nigeria. Environmental Pollution Journal. 2021; 45(3): 299–310.
- Ogundele AO, Adeoye DA, Adebayo KJ, et al. Heavy Metal Contamination from Quarry Activities in Southwest Nigeria. Environmental Monitoring and Assessment. 2019; 191(5): 327.
- Nwachukwu E, Udo AO, Mbina SO. Environmental Impact of Quarrying in Cross River State, Nigeria. Journal of Environmental Science and Toxicology. 2018; 13(1): 14–23.
- Doran JW, Zeiss MR. Soil health and sustainability: Managing the biotic component of soil quality. Applied Soil Ecology. 2000; 15(1): 3–11.
- Ololade IA, Ajayi OO. Contamination profile of major rivers along the highways in Ondo State, Nigeria. Journal of Environmental Science and Technology. 2009; 2(3): 186–194.
- Adewole MB, Adesina AO. Impact of limestone quarrying activities on soil properties in Ogun State, Nigeria. African Journal of Agricultural Research. 2011; 6(11): 2372–2377.
- Bada BS, Fagbayibgo AI. Environmental impact assessment of quarrying in Nigeria. Journal of Environmental Science and Technology. 2009; 2(2): 34–42.
- Ogbonna O, Nwosu P. Heavy metal content in soils and plants around a cement factory in Gombe State, Nigeria. Journal of Environmental Chemistry and Ecotoxicology. 2011; 3(3): 77–84.
- Singh G, Chaturvedi R. Environmental impacts of mining on biodiversity of Angul-Talcher open mining site, Orissa, India: A case study. International Journal of Environmental Sciences. 2010; 1(3): 135–142.
- Population Stat, Calabar, Nigeria population. Available online: https://populationstat.com/nigeria/calabar (accessed on 12 June 2024).
- Davidson J, Smith R, Thomas P. Geology and geomorphology of the Oban Massif region, southeastern Nigeria. Geological Society of Nigeria Press; 2019. pp. 45–47.
- Hakanson L. An ecological risk index for aquatic pollution control: A sedimentological approach. Journal of Water Research. 1980, 14(8): 975–1001.
- Qingjie G, Jun D, Yunchuan X, et al. Calculating Pollution Indices by Heavy Metals in Ecological Geochemistry Assessment and A Case Study in Parks of Beijing. Journal of China University of Geosciences. 2008; 9(3): 230–241.
- Thomilson DC, Wilson DJ, Harris CR, et al. Problem in Heavy metals in estuaries and the formation of pollution index. Helgol Wissmeeresunlter. 1980; 33(1–4): 566–575.
- USEPA (United States Environmental Protection Agency (2023), Regional Screening Levels (RSLs)–Generic Tables (2023). Available online: https://www.epa.gov/risk/regional-screening-levels-rsls-generic-tables (accessed on 6 July 2024).
- Dutch Target and Intervention Values, 2000 (the New Dutch List) Available online: http://esdat.net/Environmental%20Standards/Dutch/annexS I2000Dutch%20Environmental%20Standards.pdf (accessed on 2 June 2024).
- DEFRA and the Environment Agency. CLR11 Model Procedures for the Management of land contamination. DEFRA and the Environment Agency; 2004.
- Jeffries J. Using Soil Guideline Values. Available online: https://assets.publishing.service.gov.uk/media/5a7c94b8ed915d6969f45e46/scho0309bpqm-e-e.pdf (accessed on 2 June 2024).
- Environment Agency (2014). Soil Guideline Values for heavy metals in soil. Available online: https://www.alsenvironmental.co.uk/media-uk/pdf/datasheets/contaminated-land/als_cl_heavy-metals-guidelines-in-soil_uk_feb_17_v2.pdf (accessed on 2 June 2024).
- Ekpo FE, Nzegblue EC, Asuquo ME. A comparative study of the influence of heavy metals on soil & crops growing within quarry environment at Akamkpa, Cross River State, Nigeria. Global Journal of Agricultural Sciences. 2011; 11(1): 1–10.
- Kumar R, Singh P, Sharma N. Assessment of heavy metal contamination in quarry sites of India. Environmental Monitoring and Assessment. 2020; 192(5): 289.
Refbacks
- There are currently no refbacks.
Copyright (c) 2025 Author(s)
License URL: https://creativecommons.org/licenses/by/4.0/
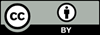
This site is licensed under a Creative Commons Attribution 4.0 International License (CC BY 4.0).
.jpg)
Beijing University of Technology, China