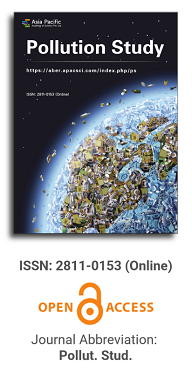
Asia Pacific Academy of Science Pte. Ltd. (APACSCI) specializes in international journal publishing. APACSCI adopts the open access publishing model and provides an important communication bridge for academic groups whose interest fields include engineering, technology, medicine, computer, mathematics, agriculture and forestry, and environment.
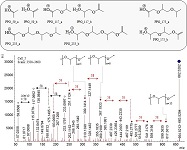
The (partial) replacement of synthetic polymers with bioplastics is due to increased production of conventional packaging plastics causing for severe environmental pollution with plastics waste. The bioplastics, however, represent complex mixtures of known and unknown (bio)polymers, fillers, plasticizers, stabilizers, flame retardant, pigments, antioxidants, hydrophobic polymers such as poly(lactic acid), polyethylene, polyesters, glycol, or poly(butylene succinate), and little is known of their chemical safety for both the environment and the human health. Polymerization reactions of bioplastics can produce no intentionally added chemicals to the bulk material, which could be toxic, as well. When polymers are used to food packing, then the latter chemicals could also migrate from the polymer to food. This fact compromises the safety for consumers, as well. The scarce data on chemical safety of bioplastics makes a gap in knowledge of their toxicity to humans and environment. Thus, development of exact analytical protocols for determining chemicals of bioplastics in environmental and food samples as well as packing polymers can only provide warrant for reliable conclusive evidence of their safety for both the human health and the environment. The task is compulsory according to legislation Directives valid to environmental protection, food control, and assessment of the risk to human health. The quantitative and structural determination of analytes is primary research task of analysis of polymers. The methods of mass spectrometry are fruitfully used for these purposes. Methodological development of exact analytical mass spectrometric tools for reliable structural analysis of bioplastics only guarantees their safety, efficacy, and quality to both humans and environment. This study, first, highlights innovative stochastic dynamics equations processing exactly mass spectrometric measurands and, thus, producing exact analyte quantification and 3D molecular and electronic structural analyses. There are determined synthetic polymers such as poly(ethylenglycol), poly(propylene glycol), and polyisoprene as well as biopolymers in bags for foodstuffs made from renewable cellulose and starch, and containing, in total within the 20,416–17,495 chemicals per sample of the composite biopolymers. Advantages of complementary employment in mass spectrometric methods and Fourier transform infrared spectroscopy is highlighted. The study utilizes ultra-high resolution electrospray ionization mass spectrometric and Fourier transform infrared spectroscopic data on biodegradable plastics bags for foodstuffs; high accuracy quantum chemical static methods, molecular dynamics; and chemometrics. There is achieved method performance |r| = 0.99981 determining poly(propylene glycol) in bag for foodstuff containing 20,416 species and using stochastic dynamics mass spectrometric formulas. The results highlight their great capability and applicability to the analytical science as well as relevance to both the fundamental research and to the industry.
Stochastic dynamics mass spectrometric and Fourier transform infrared spectroscopic structural analyses of composite biodegradable plastics
Vol 5, Issue 2, 2024
VIEWS - 274 (Abstract)
Download PDF
Abstract
The (partial) replacement of synthetic polymers with bioplastics is due to increased production of conventional packaging plastics causing for severe environmental pollution with plastics waste. The bioplastics, however, represent complex mixtures of known and unknown (bio)polymers, fillers, plasticizers, stabilizers, flame retardant, pigments, antioxidants, hydrophobic polymers such as poly(lactic acid), polyethylene, polyesters, glycol, or poly(butylene succinate), and little is known of their chemical safety for both the environment and the human health. Polymerization reactions of bioplastics can produce no intentionally added chemicals to the bulk material, which could be toxic, as well. When polymers are used to food packing, then the latter chemicals could also migrate from the polymer to food. This fact compromises the safety for consumers, as well. The scarce data on chemical safety of bioplastics makes a gap in knowledge of their toxicity to humans and environment. Thus, development of exact analytical protocols for determining chemicals of bioplastics in environmental and food samples as well as packing polymers can only provide warrant for reliable conclusive evidence of their safety for both the human health and the environment. The task is compulsory according to legislation Directives valid to environmental protection, food control, and assessment of the risk to human health. The quantitative and structural determination of analytes is primary research task of analysis of polymers. The methods of mass spectrometry are fruitfully used for these purposes. Methodological development of exact analytical mass spectrometric tools for reliable structural analysis of bioplastics only guarantees their safety, efficacy, and quality to both humans and environment. This study, first, highlights innovative stochastic dynamics equations processing exactly mass spectrometric measurands and, thus, producing exact analyte quantification and 3D molecular and electronic structural analyses. There are determined synthetic polymers such as poly(ethylenglycol), poly(propylene glycol), and polyisoprene as well as biopolymers in bags for foodstuffs made from renewable cellulose and starch, and containing, in total within the 20,416–17,495 chemicals per sample of the composite biopolymers. Advantages of complementary employment in mass spectrometric methods and Fourier transform infrared spectroscopy is highlighted. The study utilizes ultra-high resolution electrospray ionization mass spectrometric and Fourier transform infrared spectroscopic data on biodegradable plastics bags for foodstuffs; high accuracy quantum chemical static methods, molecular dynamics; and chemometrics. There is achieved method performance |r| = 0.99981 determining poly(propylene glycol) in bag for foodstuff containing 20,416 species and using stochastic dynamics mass spectrometric formulas. The results highlight their great capability and applicability to the analytical science as well as relevance to both the fundamental research and to the industry.
Keywords
References
1. Zhang H, Su Z, Wang X. Starch-Based Rehealable and Degradable Bioplastic Enabled by Dynamic Imine Chemistry. ACS Sustainable Chemistry & Engineering. 2022; 10(26): 8650-8657. doi: 10.1021/acssuschemeng.2c02537
2. Shafqat A, Al-Zaqri N, Tahir A, et al. Synthesis and characterization of starch based bioplatics using varying plant-based ingredients, plasticizers and natural fillers. Saudi Journal of Biological Sciences. 2021; 28(3): 1739-1749. doi: 10.1016/j.sjbs.2020.12.015
3. Rizzarelli P, Leanza M, Rapisarda M. Investigations into the characterization, degradation, and applications of biodegradable polymers by mass spectrometry. Mass Spectrometry Reviews. Published online November 28, 2023. doi: 10.1002/mas.21869
4. Chia HN, Wu BM. Recent advances in 3D printing of biomaterials. Journal of Biological Engineering. 2015; 9(1). doi: 10.1186/s13036-015-0001-4
5. Abed SH, Shamkhi AF, Heydaryan K, et al. Sol-gel Pechini preparation of CuEr2TiO6 nanoparticles as highly efficient photocatalyst for visible light degradation of acid red 88. Ceramics International. 2024; 50(13): 24096-24102. doi: 10.1016/j.ceramint.2024.04.141
6. Chen X, Yan S, Wang H, et al. Aerobic oxidation of starch catalyzed by isopolyoxovanadate Na4Co(H2O)6V10O28. Carbohydrate Polymers. 2015; 117: 673-680. doi: 10.1016/j.carbpol.2014.10.066
7. Kiani Z, Mirjalili S, Heydaryan K, et al. Harmonizing nature and nanotechnology: Phytoextract-mediated synthesis of Ag-doped ZnO nanoparticles using Lavandula stoechas extract for environmental and biomedical applications. Journal of Drug Delivery Science and Technology. 2024; 96: 105708. doi: 10.1016/j.jddst.2024.105708
8. Khanjari Z, Chamani E, Heydaryan K, et al. Unlocking the therapeutic potential: Green synthesized zinc oxide/silver nanoparticles from Sophora pachycarpa for anticancer activity, gene expression analysis, and antibacterial applications. Materials Today Communications. 2024; 39: 109214. doi: 10.1016/j.mtcomm.2024.109214
9. Ghazi RA, Jasim AS, Heydaryan K, et al. Biosynthesis of Ag-Doped CuO Nanoparticles Using Heracleum persicum Extract for Enhanced Antibacterial and Photocatalytic Dye Degradation Properties. Plasmonics. 2024. doi: 10.1007/s11468-024-02298-1
10. Kashi MA, Heydaryan K, Khojasteh H, et al. Green Synthesis of Ag NPs/rGO Nanocomposite for Use as a Non-enzymatic Sensor of H2O2. Plasmonics. 2024. doi: 10.1007/s11468-024-02330-4
11. Khojasteh H, Heydaryan K, Aspoukeh P, et al. Characterization and Application of CuO Nanoparticles in Gelatin-Glycerol Coatings for Enhanced Shelf Life of Strawberries. Plasmonics. 2024. doi: 10.1007/s11468-024-02345-x
12. Abed SH, Madhi RA, Heydaryan K, et al.Shamkhi A. Green synthesis of gold-doped ZnFe2O4 nanoparticles using Crataegus monogyna leaf extract: characterization, antibacterial, and efficient degradation of methylene blue and eriochrome black T pollutants. Biomass Conversion and Biorefinery. 2024. doi: 10.1007/s13399-024-05337-3
13. Zimmermann L, Dombrowski A, Völker C, et al. Are bioplastics and plant-based materials safer than conventional plastics? In vitro toxicity and chemical composition. Environment International. 2020; 145: 106066. doi: 10.1016/j.envint.2020.106066
14. Binda G, Costa M, Supraha L, et al. Untangling the role of biotic and abiotic ageing of various environmental plastics toward the sorption of metals. Science of The Total Environment. 2023; 893: 164807. doi: 10.1016/j.scitotenv.2023.164807
15. Anna V, Rimma S, Lev O, et al. GC determination of N-nitrosamines by supersonic molecular beam MS equipped with triple quadrupole analyzer, GC/SMB/QQQ/MS. Analytica Chimica Acta. 2011; 685(2): 162-169. doi: 10.1016/j.aca.2010.11.026
16. Zimmermann L, Dierkes G, Ternes TA, et al. Benchmarking the in Vitro Toxicity and Chemical Composition of Plastic Consumer Products. Environmental Science & Technology. 2019; 53(19): 11467-11477. doi: 10.1021/acs.est.9b02293
17. Regulation (EC) No 1935/2004 of the European Parliament and the Council. On materials and articles intended to come into contact with food and repealing Directives 80/590/EEC and 89/109/EEC. Official Journal of the European Union L338/4. 2004.
18. European Commission. European Commission (2011) Commission regulation (EU) No 10/2011 of 14 January 2011 on plastic materials and articles intended to come into contact with food. Official Journal of the European Union L12/1. 2011.
19. Bos TS, Pirok BWJ, Karlson L, et al. Fingerprinting of hydroxy propyl methyl cellulose by comprehensive two-dimensional liquid chromatography-mass spectrometry of monomers resulting from acid hydrolysis. Journal of Chromatography A. 2024; 1722: 464874. doi: 10.1016/j.chroma.2024.464874
20. Kumar A, Dutta D, Kalita D, et al. Extraction, physicochemical and structural characterisation of palm grass leaf fibres for sustainable and cleaner production of textile and allied cellulosic applications. Journal of Cleaner Production. 2024; 448: 141733. doi: 10.1016/j.jclepro.2024.141733
21. Xue P, Liu M, Yang H, et al. Mechanism study on pyrolysis interaction between cellulose, hemicellulose, and lignin based on photoionization time-of-flight mass spectrometer (PI-TOF-MS) analysis. Fuel. 2023; 338: 127276. doi: 10.1016/j.fuel.2022.127276
22. Chen D, Cen K, Zhuang X, et al. Insight into biomass pyrolysis mechanism based on cellulose, hemicellulose, and lignin: Evolution of volatiles and kinetics, elucidation of reaction pathways, and characterization of gas, biochar and bio‐oil. Combustion and Flame. 2022; 242: 112142. doi: 10.1016/j.combustflame.2022.112142
23. Zhang D, Loomer M, Gamez G. Quantitative analysis of biopolymers in lignocellulosic biomass feedstocks via laser-assisted micro-pyrolysis flowing atmospheric-pressure afterglow high-resolution ambient mass spectrometry. Talanta. 2024; 268: 125333. doi: 10.1016/j.talanta.2023.125333
24. Ruggero F, Carretti E, Gori R, et al. Monitoring of degradation of starch-based biopolymer film under different composting conditions, using TGA, FTIR and SEM analysis. Chemosphere. 2020; 246: 125770. doi: 10.1016/j.chemosphere.2019.125770
25. Tolonen LK, Juvonen M, Niemelä K, et al. Supercritical water treatment for cello-oligosaccharide production from microcrystalline cellulose. Carbohydrate Research. 2015; 401: 16-23. doi: 10.1016/j.carres.2014.10.012
26. Osorio J, Aznar M, Nerín C, et al. Comparison of LC-ESI, DART, and ASAP for the analysis of oligomers migration from biopolymer food packaging materials in food (simulants). Analytical and Bioanalytical Chemistry. 2021; 414(3): 1335-1345. doi: 10.1007/s00216-021-03755-0
27. Golon A, González FJ, Dávalos JZ, et al. Investigating the Thermal Decomposition of Starch and Cellulose in Model Systems and Toasted Bread Using Domino Tandem Mass Spectrometry. Journal of Agricultural and Food Chemistry. 2013; 61(3): 674-684. doi: 10.1021/jf302135k
28. Ghiulai R, Galusca M, Sisu I, et al. High resolution mass spectrometric characterization of amino linked oligosaccharides—a preliminary study. Open Chemistry. 2013; 11(8): 1309-1319. doi: 10.2478/s11532-013-0265-9
29. Jiang J, Wang X. Adsorption of Hg(II) ions from aqueous solution by thiosemicarbazide-modified cellulose adsorbent. BioResources. 2019; 14(2): 4670-4695. doi: 10.15376/biores.14.2.4670-4695
30. Ivanova B. Special Issue with Research Topics on “Recent Analysis and Applications of Mass Spectra on Biochemistry.” International Journal of Molecular Sciences. 2024; 25(4): 1995. doi: 10.3390/ijms25041995
31. Wang Z, Liu PK, Li L. A Tutorial Review of Labeling Methods in Mass Spectrometry-Based Quantitative Proteomics. ACS Measurement Science Au. 2024. doi: 10.1021/acsmeasuresciau.4c00007
32. Calvano CD, Cataldi TRI, Kögel JF, et al. Structural Characterization of Neutral Saccharides by Negative Ion MALDI Mass Spectrometry Using a Superbasic Proton Sponge as Deprotonating Matrix. Journal of the American Society for Mass Spectrometry. 2017; 28(8): 1666-1675. doi: 10.1007/s13361-017-1679-y
33. Wesdemiotis C. Multidimensional Mass Spectrometry of Synthetic Polymers and Advanced Materials. Angewandte Chemie International Edition. 2017; 56(6): 1452-1464. doi: 10.1002/anie.201607003
34. Wesdemiotis C, Williams‐Pavlantos KN, Keating AR, et al. Mass spectrometry of polymers: A tutorial review. Mass Spectrometry Reviews. 2023; 43(3): 427-476. doi: 10.1002/mas.21844
35. Lattimer R. Tandem mass-spectrometry of poly(ethylene glycol) proton-attachment and deuteron-attachment ions. International Journal of Mass Spectrometry. 1992; 116: 23-36. doi: 10.1016/0168-1176(92)80017-U
36. Li X, Guo L, Casiano-Maldonado M, et al. Top-Down Multidimensional Mass Spectrometry Methods for Synthetic Polymer Analysis. Macromolecules. 2011; 44(12): 4555-4564. doi: 10.1021/ma200542p
37. Buck-Wiese H, Fanuel M, Liebeke M, et al. Discrimination of β-1,4- and β-1,3-Linkages in Native Oligosaccharides via Charge Transfer Dissociation Mass Spectrometry. Journal of the American Society for Mass Spectrometry. 2020; 31(6): 1249-1259. doi: 10.1021/jasms.0c00087
38. Wesdemiotis C, Solak N, Polce MJ, et al. Fragmentation pathways of polymer ions. Mass Spectrometry Reviews. 2011; 30(4): 523-559. doi: 10.1002/mas.20282
39. Stephens CH, Shrestha B, Morris HR, et al. Minimally invasive monitoring of cellulose degradation by desorption electrospray ionization and laser ablation electrospray ionization mass spectrometry. The Analyst. 2010; 135(9): 2434. doi: 10.1039/c0an00155d
40. Pfenninger A, Karas M, Finke B, Stahl B. Structural analysis of underivatized neutral human milk oligosaccharides in the negative ion mode by nano-electrospray MSn (Part 2). Journal of the American Society for Mass Spectrometry. 2002; 13: 1341-1348. doi: 10.1016/S1044-0305(02)00646-3
41. Montaudo G, Samperi F, Montaudo MS. Characterization of synthetic polymers by MALDI-MS. Progress in Polymer Science. 2006; 31(3): 277-357. doi: 10.1016/j.progpolymsci.2005.12.001
42. Schulz H, Baranska M. Identification and quantification of valuable plant substances by IR and Raman spectroscopy. Vibrational Spectroscopy. 2007; 43(1): 13-25. doi: 10.1016/j.vibspec.2006.06.001
43. Alves APP, de Oliveira LPZ, Castro AAN, et al. The structure of different cellulosic fibres characterized by Raman spectroscopy. Vibrational Spectroscopy. 2016; 86: 324-330. doi: 10.1016/j.vibspec.2016.08.007
44. Tallarico S, Bonacci S, Mancuso S, et al. Quali-quantitative monitoring of chemocatalytic cellulose conversion into lactic acid by FT-NIR spectroscopy. Spectrochimica Acta Part A: Molecular and Biomolecular Spectroscopy. 2021; 250: 119367. doi: 10.1016/j.saa.2020.119367
45. Kotatha D, Rungrodnimitchai S. Synthesis and Characterization of Nanofiber of Oxidized Cellulose from Nata De Coco. International Journal of Chemical Engineering. 2018; 2018: 1-12. doi: 10.1155/2018/2787035
46. Document 31996L0023: Commision Decision of 12.08.2002 implementing Council Directive 96/23/EC concerning the performance of analytical methods and the interpretation of results; notified under document number C(2002) 3044; Text with EEA relevance 2002/657/EC. Official Journal of the European Union L 221/8. 2022.
47. Ivanova B, Spiteller M. Electrospray ionization stochastic dynamic mass spectrometric 3D structural analysis of ZnII-ion containing complexes in solution. Inorganic and Nano-Metal Chemistry. 2021; 52(12): 1407-1429. doi: 10.1080/24701556.2021.1956963
48. Ivanova B, Tsalev D, Arnaudov M. Validation of reducing-difference procedure for the interpretation of non-polarized infrared spectra of n-component solid mixtures. Talanta. 2006; 69(4): 822-828. doi: 10.1016/j.talanta.2005.11.026
49. Ivanova B. Stochastic Dynamic Mass Spectrometric Quantitative and Structural Analyses of Pharmaceutics and Biocides in Biota and Sewage Sludge. International Journal of Molecular Sciences. 2023; 24(7): 6306. doi: 10.3390/ijms24076306
50. Ivanova B, Spiteller M. Stochastic dynamic mass spectrometric quantification of steroids in mixture—Part II. Steroids. 2020; 164: 108750. doi: 10.1016/j.steroids.2020.108750
51. Ivanova B, Spiteller M. Stochastic Dynamic Mass Spectrometric Approach to Quantify Reserpine in Solution. Analytical Chemistry Letters. 2020; 10(6): 703-721. doi: 10.1080/22297928.2020.1865834
52. Ivanova B, Spiteller M. Chapter 1: Mass spectrometric and quantum chemical treatments of molecular and ionic interactions of a flavonoid-O-glycoside—A stochastic dynamic approach. In: Advances in Chemistry Research. NOVA Science Publishers, New York; 2022. pp. 1-126.
53. Ivanova B, Spiteller M. Stochastic Dynamic Electrospray Ionization Mass Spectrometric Quantitative Analysis of Metronidazole in Human Urine. Analytical Chemistry Letters. 2022; 12(3): 322-348. doi: 10.1080/22297928.2022.2086822
54. Ivanova B, Spiteller M. Exact Quantifying of Mass Spectrometric Variable Intensity of Analyte Peaks with Respect to Experimental Conditions of Measurements—A Stochastic Dynamic Approach. Analytical Chemistry Letters. 2022; 12(5): 542-561. doi: 10.1080/22297928.2022.2142844
55. Ivanova B, Spiteller M. Stochastic dynamic ultraviolet photofragmentation and high collision energy dissociation mass spectrometric kinetics of triadimenol and sucralose. Environmental Science and Pollution Research. 2022; 30(12): 32348-32370. doi: 10.1007/s11356-022-24259-z
56. Ivanova B, Spiteller M. Stochastic dynamic quantitative and 3D structural matrix assisted laser desorption/ionization mass spectrometric analyses of mixture of nucleosides. Journal of Molecular Structure. 2022; 1260: 132701. doi: 10.1016/j.molstruc.2022.132701
57. Ivanova B. 3D structural analysis of alanyl-containing peptides of silac-proteomics—an approach to stochastic dynamics. SSNR eJournal. 2023. doi: 10.2139/ssrn.4498960
58. Upadyshev M, Ivanova B, Motyleva S. Mass Spectrometric Identification of Metabolites after Magnetic-Pulse Treatment of Infected Pyrus communis L. Microplants. International Journal of Molecular Sciences. 2023; 24(23): 16776. doi: 10.3390/ijms242316776
59. Ivanova B. Stochastic dynamics mass spectrometric structural analysis of poly(methyl methacrylate). Universal Journal of Carbon Research. 2024: 60-88. doi: 10.37256/ujcr.2120244425
60. Zimmermann L, Zimmermann L, Dombrowski A, et al. Raw data for “Are bioplastics and plant-based materials safer than conventional plastics? In vitro toxicity and chemical composition”. Available online: https://zenodo.org/records/4004763 (accessed on 27 August 2020).
61. Centexbel. Gudelines for data-analysis, calibration, and prediction. SAFIR project WP3/SMT4-CT96-2136. VTT Rakennus; 2002.
62. Villegas-Camacho O, Alejo-Eleuterio R, Francisco-Valencia I, et al. FTIR-Plastics: A Fourier Transform Infrared Spectroscopy dataset for the six most prevalent industrial plastic polymers. Data in Brief. 2024; 55: 110612. doi: 10.1016/j.dib.2024.110612
63. Frisch M, Trucks G, Schlegel H, et al. Gaussian 09, 98. Gaussian, Inc., Pittsburgh, Wallingford CT; 2009.
64. Aidas K, Angeli C, Bak K et al. The Dalton quantum chemistry program system. Wiley Interdisciplinary Reviews: Computational Molecular Science. 2014; 4: 269-284. doi: 10.1002/wcms.1172
65. Gordon MS, Schmidt MW. Advances in electronic structure theory. Theory and Applications of Computational Chemistry. 2005; 1167-1189. doi: 10.1016/b978-044451719-7/50084-6
66. Nielsen A, Holder A. Gauss View 5.0, User's Reference. Pittsburgh GausView03 Program Package. GAUSSIAN Inc.; 2009.
67. Burkert U, Allinger N. Molecular mechanics in ACS Monograph 177. American Chemical Society, Washington D.C.; 1982.
68. Allinger NL. Conformational analysis. 130. MM2. A hydrocarbon force field utilizing V1 and V2 torsional terms. Journal of the American Chemical Society. 1977; 99(25): 8127-8134. doi: 10.1021/ja00467a001
69. Kelley C. Iterative Methods for optimization. SIAM Front. Appl. Mathematics. 2009; 18.
70. Otto M. Chemometrics, 3rd ed. Wiley, Weinheim; 2017.
71. Apache OpenOffice. Available online: http://de.openoffice.org (accessed on 3 May 2024).
72. Madsen K, Nielsen H, Tingleff T. Informatics and mathematical modelling, 2nd ed. DTU Press; 2004.
73. Miller J, Miller J. Statistics and chemometrics for analytical chemistry. Pentice Hall, London; 1988.
74. Taylor J. Quality assurance of chemical measurements. Lewis Publishers, Inc.; 1987.
75. Schroee G, Trenkler D. Exact and randomization distributions of Kolmogorov-Smirnov tests two or three samples. Computational Statistics & Data Analysis. 1995; 20: 185-202. doi: 10.1016/0167-9473(94)00040-P
76. Fay MP, Proschan MA. Wilcoxon-Mann-Whitney or t-test? On assumptions for hypothesis tests and multiple interpretations of decision rules. Statistics Surveys. 2010; 4. doi: 10.1214/09-ss051
77. Freidlin B, Gastwirth JL. Should the Median Test be Retired from General Use? The American Statistician. 2000; 54(3): 161-164. doi: 10.1080/00031305.2000.10474539
78. Kunov-Kruse AJ, Riisager A, Saravanamurugan S, et al. Revisiting the Brønsted acid catalysed hydrolysis kinetics of polymeric carbohydrates in ionic liquids by in situ ATR-FTIR spectroscopy. Green Chemistry. 2013; 15(10): 2843. doi: 10.1039/c3gc41174e
79. Jackson A, Yates H, Scrivens J, et al. The application of matrix-assisted laser desorption/ionization combined with collision-induced dissociation to the analysis of synthetic polymers. Rapid Communications Mass Spectrometry. 1996; 10: 1668-1674. doi: 10.1002/(SICI)1097-0231(199610)10:13<1668::AID-RCM703>3.0.CO;2-I
80. Scrivens J, Jackson A, Yates H, et al. The effect of the variation of cation in the matrix-assisted laser desorption/ionisation collision induced dissociation (MALDI-CID) spectra of oligomeric systems. International Journal of Mass Spectrometry. 1997; 165: 363-375. doi: 10.1016/S0168-1176(97)00239-5
81. Jackson AT, Green MR, Bateman RH. Generation of end‐group information from polyethers by matrix‐assisted laser desorption/ionisation collision‐induced dissociation mass spectrometry. Rapid Communications in Mass Spectrometry. 2006; 20(23): 3542-3550. doi: 10.1002/rcm.2773
82. Lattimer R. Tandem mass-spectrometry of lithium-attachment ions from polyglycols. Journal of the American Society for Mass Spectrometry. 1992; 3: 225-234. doi: 10.1016/1044-0305(92)87006-K
83. Lattimer R. Tandem mass-spectrometry of poly(ethylene glycol) lithium-attachment ions. Journal of the American Society for Mass Spectrometry. 1994; 5: 1072-1080. doi: 10.1016/1044-0305(94)85068-2
84. Selby T, Wesdemiotis C, Lattimer R. Dissociation characteristics of [M-X]-ions (X = H, Li, K) from linear and cyclic polyglycols. Journal of the American Society for Mass Spectrometry. 1994; 5: 1081-1092. doi: 10.1016/1044-0305(94)85069-0
85. Lattimer R. Mass spectral analysis of low-temperature pyrolysis products from poly(ethylene glycol). Journal of Analytical and Applied Pyrolysis. 2000; 56: 61-78. doi: 10.1016/S0165-2370(00)00074-7
86. Scionti V, Katzenmeyer BC, Erdem NS, et al. Interfacing Multistage Mass Spectrometry with Liquid Chromatography or Ion Mobility Separation for Synthetic Polymer Analysis. European Journal of Mass Spectrometry. 2012; 18(2): 113-137. doi: 10.1255/ejms.1175
87. D’Alexandri FL, Gozzo FC, Eberlin MN, et al. Electrospray ionization mass spectrometry analysis of polyisoprenoid alcohols via Li+ cationization. Analytical Biochemistry. 2006; 355(2): 189-200. doi: 10.1016/j.ab.2006.06.014
88. Snyder SR, Wesdemiotis C. Elucidation of Low Molecular Weight Polymers in Vehicular Engine Deposits by Multidimensional Mass Spectrometry. Energy & Fuels. 2020; 35(2): 1691-1700. doi: 10.1021/acs.energyfuels.0c02702
89. Voorhees K, Baugh S, Stevenson D. An investigation of the thermal degradation of poly(ethylene glycol). Journal of Analytical and Applied Pyrolysis. 1994; 30: 47-57. doi: 10.1016/0165-2370(94)00803-5
90. Endres KJ, Dilla RA, Becker ML, et al. Poly(ethylene glycol) Hydrogel Crosslinking Chemistries Identified via Atmospheric Solids Analysis Probe Mass Spectrometry. Macromolecules. 2021; 54(17): 7754-7764. doi: 10.1021/acs.macromol.1c00765
91. Chen H, He M, Pei J, et al. Quantitative Analysis of Synthetic Polymers Using Matrix-Assisted Laser Desorption/Ionization Time-of-Flight Mass Spectrometry. Analytical Chemistry. 2003; 75(23): 6531-6535. doi: 10.1021/ac0344034
92. Okuno S, Wada Y, Arakawa R. Quantitative analysis of polypropyleneglycol mixtures by desorption/ionization on porous silicon mass spectrometry. International Journal of Mass Spectrometry. 2005; 241(1): 43-48. doi: 10.1016/j.ijms.2004.10.022
93. Boulos S, Nyström L. UPLC-MS/MS investigation of β-glucan oligosaccharide oxidation. The Analyst. 2016; 141(24): 6533-6548. doi: 10.1039/c6an01125j
94. Vuong TV, Vesterinen AH, Foumani M, et al. Xylo- and cello-oligosaccharide oxidation by gluco-oligosaccharide oxidase from Sarocladium strictumand variants with reduced substrate inhibition. Biotechnology for Biofuels. 2013; 6(1). doi: 10.1186/1754-6834-6-148
95. Dallinga JW, Heerma W. Reaction mechanism and fragment ion structure determination of deprotonated small oligosaccharides, studied by negative ion fast atom bombardment (tandem) mass spectrometry. Biological Mass Spectrometry. 1991; 20(4): 215-231. doi: 10.1002/bms.1200200410
96. Swanson KD, Worth AL, Glish GL. Use of an Open Port Sampling Interface Coupled to Electrospray Ionization for the On-Line Analysis of Organic Aerosol Particles. Journal of the American Society for Mass Spectrometry. 2017; 29(2): 297-303. doi: 10.1007/s13361-017-1776-y
97. Kraska U, Micheel F. Synthetische polysaccharide mit D-glucose und 3,6-anhydro-D-glucoseresten. Carbohydrate Research. 1976; 49: 195-199. doi: 10.1016/S0008-6215(00)83137-7
98. Kochetkov NK, Chizhov OS, Zolotarev BM. Mass-spectrometric study of carbohydrates. Chemistry of Natural Compounds. 1966; 2(3): 120-124. doi: 10.1007/bf01134228
99. Dufour A, Weng J, Jia L, et al. Revealing the chemistry of biomass pyrolysis by means of tunable synchrotron photoionisation-mass spectrometry. RSC Advances. 2013; 3(14): 4786. doi: 10.1039/c3ra40486b
100. Kim J, Yun E, Yu S, et al. Different Levels of Skin Whitening Activity among 3,6-Anhydro-l-galactose, Agarooligosaccharides, and Neoagarooligosaccharides. Marine Drugs. 2017; 15(10): 321. doi: 10.3390/md15100321
101. Chen C, Chen J, Geng Z, et al. Regioselectivity of oxidation by a polysaccharide monooxygenase from Chaetomium thermophilum. Biotechnology for Biofuels. 2018; 11(1). doi: 10.1186/s13068-018-1156-2
102. Fanuel M, Garajova S, Ropartz D, et al. The Podospora anserina lytic polysaccharide monooxygenase PaLPMO9H catalyzes oxidative cleavage of diverse plant cell wall matrix glycans. Biotechnology for Biofuels. 2017; 10(1). doi: 10.1186/s13068-017-0749-5
103. Bennati-Granier C, Garajova S, Champion C, et al. Substrate specificity and regioselectivity of fungal AA9 lytic polysaccharide monooxygenases secreted by Podospora anserina. Biotechnology for Biofuels. 2015; 8(1). doi: 10.1186/s13068-015-0274-3
104. Sun P, Frommhagen M, Kleine Haar M, et al. Mass spectrometric fragmentation patterns discriminate C1- and C4-oxidised cello-oligosaccharides from their non-oxidised and reduced forms. Carbohydrate Polymers. 2020; 234: 115917. doi: 10.1016/j.carbpol.2020.115917
105. Frommhagen M, van Erven G, Sanders M, et al. RP-UHPLC-UV-ESI-MS/MS analysis of LPMO generated C4-oxidized gluco-oligosaccharides after non-reductive labeling with 2-aminobenzamide. Carbohydrate Research. 2017; 448: 191-199. doi: 10.1016/j.carres.2017.03.006
106. Isaksen T, Westereng B, Aachmann FL, et al. A C4-oxidizing Lytic Polysaccharide Monooxygenase Cleaving Both Cellulose and Cello-oligosaccharides. Journal of Biological Chemistry. 2014; 289(5): 2632-2642. doi: 10.1074/jbc.m113.530196
107. Silva C de OG, Teixeira TS, Rodrigues KB, et al. Combination of MALDI-TOF MS and UHPLC-ESI-MS for the characterization of lytic polysaccharide monooxygenase activity. Analytical Methods. 2020; 12(2): 149-161. doi: 10.1039/c9ay01774g
108. Sun P, Laurent CVFP, Boerkamp VJP, et al. Regioselective C4 and C6 Double Oxidation of Cellulose by Lytic Polysaccharide Monooxygenases. ChemSusChem. 2021; 15(2). doi: 10.1002/cssc.202102203
109. Mudedla SK, Vuorte M, Veijola E, et al. Effect of oxidation on cellulose and water structure: a molecular dynamics simulation study. Cellulose. 2021; 28(7): 3917-3933. doi: 10.1007/s10570-021-03751-8
110. Westereng B, Arntzen MØ, Aachmann FL, et al. Simultaneous analysis of C1 and C4 oxidized oligosaccharides, the products of lytic polysaccharide monooxygenases acting on cellulose. Journal of Chromatography A. 2016; 1445: 46-54. doi: 10.1016/j.chroma.2016.03.064
111. Domon B, Costello CE. A systematic nomenclature for carbohydrate fragmentations in FAB-MS/MS spectra of glycoconjugates. Glycoconjugate Journal. 1988; 5(4): 397-409. doi: 10.1007/bf01049915
112. Stephens E, Maslen SL, Green LG, et al. Fragmentation Characteristics of Neutral N-Linked Glycans Using a MALDI-TOF/TOF Tandem Mass Spectrometer. Analytical Chemistry. 2004; 76(8): 2343-2354. doi: 10.1021/ac030333p
113. Spina E, Sturiale L, Romeo D, et al. New fragmentation mechanisms in matrix‐assisted laser desorption/ionization time‐of‐flight/time‐of‐flight tandem mass spectrometry of carbohydrates. Rapid Communications in Mass Spectrometry. 2004; 18(4): 392-398. doi: 10.1002/rcm.1350
114. Moseley HNB, Rocca-Serra P, Salek RM, et al. InChI isotopologue and isotopomer specifications. Journal of Cheminformatics. 2024; 16(1). doi: 10.1186/s13321-024-00847-8
115. Romson J, Emmer Å. Mass calibration options for accurate electrospray ionization mass spectrometry. International Journal of Mass Spectrometry. 2021; 467: 116619. doi: 10.1016/j.ijms.2021.116619
116. Qiu L, Cooks RG. Pitfalls in Using Isotopic Distributions for Structural Interpretation by Mass Spectrometry. Journal of the American Society for Mass Spectrometry. 2024; 35(3): 642-645. doi: 10.1021/jasms.3c00457
117. Claesen J, Rockwood A, Gorshkov M, et al. The isotope distribution: A rose with thorns. Mass Spectrometry Reviews. 2023. doi: 10.1002/mas.21820
118. Satoh A. Introduction to practice of molecular simulation: Molecular dynamics, Monte Carlo, Brownian dynamics, lattice Boltzmann, dissipative particle dynamics. In: Elsevier insights Series. Elsevier, Amsterdam; 2011.
119. Ivanova B, Spiteller M. Experimental and theoretical mass spectrometric quantification of diffusion parameters and 3D structural determination of ions of L-tryptophyl-l-tryptophan in electrospray ionization conditions in positive operation mode. Journal of Molecular Structure. 2018; 1173: 848-864. doi: 10.1016/j.molstruc.2018.07.055
120. Ivanova B, Spiteller M. A mass spectrometric stochastic dynamic diffusion approach to selective quantitative and 3D structural analyses of native cyclodextrins by electrospray ionization and atmospheric pressure chemical ionization methods. Bioorganic Chemistry. 2019; 93: 103308. doi: 10.1016/j.bioorg.2019.103308
121. Ivanova B, Spiteller M. Mass spectrometric stochastic dynamic 3D structural analysis of mixture of steroids in solution – Experimental and theoretical study. Steroids. 2022; 181: 109001. doi: 10.1016/j.steroids.2022.109001
Refbacks
- There are currently no refbacks.
Copyright (c) 2024 Bojidarka Ivanova
License URL: https://creativecommons.org/licenses/by/4.0/
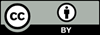
This site is licensed under a Creative Commons Attribution 4.0 International License (CC BY 4.0).
.jpg)
Prof. Hongxing Dai
Beijing University of Technology, China