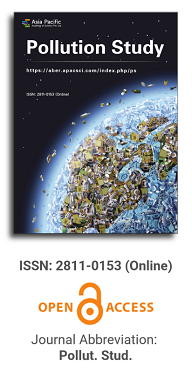
Asia Pacific Academy of Science Pte. Ltd. (APACSCI) specializes in international journal publishing. APACSCI adopts the open access publishing model and provides an important communication bridge for academic groups whose interest fields include engineering, technology, medicine, computer, mathematics, agriculture and forestry, and environment.
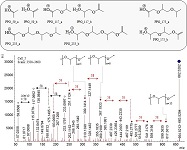
The (partial) replacement of synthetic polymers with bioplastics is due to increased production of conventional packaging plastics causing for severe environmental pollution with plastics waste. The bioplastics, however, represent complex mixtures of known and unknown (bio)polymers, fillers, plasticizers, stabilizers, flame retardant, pigments, antioxidants, hydrophobic polymers such as poly(lactic acid), polyethylene, polyesters, glycol, or poly(butylene succinate), and little is known of their chemical safety for both the environment and the human health. Polymerization reactions of bioplastics can produce no intentionally added chemicals to the bulk material, which could be toxic, as well. When polymers are used to food packing, then the latter chemicals could also migrate from the polymer to food. This fact compromises the safety for consumers, as well. The scarce data on chemical safety of bioplastics makes a gap in knowledge of their toxicity to humans and environment. Thus, development of exact analytical protocols for determining chemicals of bioplastics in environmental and food samples as well as packing polymers can only provide warrant for reliable conclusive evidence of their safety for both the human health and the environment. The task is compulsory according to legislation Directives valid to environmental protection, food control, and assessment of the risk to human health. The quantitative and structural determination of analytes is primary research task of analysis of polymers. The methods of mass spectrometry are fruitfully used for these purposes. Methodological development of exact analytical mass spectrometric tools for reliable structural analysis of bioplastics only guarantees their safety, efficacy, and quality to both humans and environment. This study, first, highlights innovative stochastic dynamics equations processing exactly mass spectrometric measurands and, thus, producing exact analyte quantification and 3D molecular and electronic structural analyses. There are determined synthetic polymers such as poly(ethylenglycol), poly(propylene glycol), and polyisoprene as well as biopolymers in bags for foodstuffs made from renewable cellulose and starch, and containing, in total within the 20,416–17,495 chemicals per sample of the composite biopolymers. Advantages of complementary employment in mass spectrometric methods and Fourier transform infrared spectroscopy is highlighted. The study utilizes ultra-high resolution electrospray ionization mass spectrometric and Fourier transform infrared spectroscopic data on biodegradable plastics bags for foodstuffs; high accuracy quantum chemical static methods, molecular dynamics; and chemometrics. There is achieved method performance |r| = 0.99981 determining poly(propylene glycol) in bag for foodstuff containing 20,416 species and using stochastic dynamics mass spectrometric formulas. The results highlight their great capability and applicability to the analytical science as well as relevance to both the fundamental research and to the industry.
Use of biochar as an alternative material for the treatment of polluted wastewater
Vol 5, Issue 1, 2024
Download PDF
Abstract
Biochar is the product of biomass decomposition, whose physicochemical characteristics are associated with its origin and the combustion method used. Among these properties, the surface area, the formation of macro and micropores, and the presence of functional groups stand out. Due to these characteristics, biochar becomes an alternative material with high adsorption capacity of toxic compounds present in contaminated wastewater. This work provides information on the generation mechanisms of biochar and how they interfere in its physicochemical characteristics. It also describes the parameters involved in the pollutant removal processes and mentions the treatments under which biochar can be subjected to improve its adsorption capacity. Finally, the possible uses or the appropriate final disposal of biochar in order to contribute to the circular economy strategy are indicated.
Keywords
References
- Spain: Ministry of Defense. Spanish Institute for Strategic Studies, Cuadernos de Estrategia 186 Water: source of conflict or cooperation? Spain: Ministry of Defense; 2017.
- Espigares García M, Pérez López JA. Wastewater: The wasted resource (Spanish). Universidad de Granada; 1990.
- Preston RJ, Skare JA, Aardema MJ. A review of biomonitoring studies measuring genotoxicity in humans exposed to hair dyes. Mutagenesis. 2010; 2(1): 17-23. doi: 10.1093/mutage/gep044
- Klaunig JE, Kamendulis LM, Hocevar BA. Oxidative stress and oxidative damage in carcinogenesis. Toxicologic Pathology. 2010; 38(1): 96-109. doi: 10.1177/0192623309356453
- Javaid R, Qazi UY. Catalytic Oxidation Process for the Degradation of Synthetic Dyes: An Overview. International Journal of Environmental Research and Public Health. 2019; 16(11): 2066. doi: 10.3390/ijerph16112066
- Pagalan Jr E, Sebron M, Gomez S, et al. Activated carbon from spent coffee grounds as an adsorbent for treatment of water contaminated by aniline yellow dye. Industrial Crops and Products. 2020; 145: 111953. doi: 10.1016/j.indcrop.2019.111953
- De la peña ME, Ducci J, Zamora V. Wastewater treatment in Mexico. Inter-American Development Bank, Mexico; 2013.
- Valdez EC, Vásquez AB. Engineering of wastewater treatment and disposal systems (Spanish). Mexico: Fundación ICA; 2003.
- Anjaneyulu Y, Sreedhara Chary N, Samuel Suman Raj D. Decolourization of Industrial Effluents—Available Methods and Emerging Technologies—A Review. Reviews in Environmental Science and Bio/Technology. 2005; 4(4): 245-273. doi: 10.1007/s11157-005-1246-z
- Cortazar Martínez A, González Ramírez CA, Coronel Olivares C, et al. Biotechnology applied to the degradation of textile industry dyes. Biotechnology applied to the degradation of textile industry dyes. Universidad y Ciencia. 2012; 28(2): 187-199.
- Barrios-Ziolo LF, Gaviria-Restrepo LF, Agudelo EA, et alTechnologies for the Removal of Dyes and Pigments from Wastewater (Spanish). DYNA. 2015; 82(191): 118-126. doi: 10.15446/dyna.v82n191.42924
- Choudhary M, Kumar R, Neogi S. Activated biochar derived from Opuntia ficus-indica for the efficient adsorption of malachite green dye, Cu+2 and Ni+2 from water. Journal of Hazardous Materials. 2020; 392: 122441. doi: 10.1016/j.jhazmat.2020.122441
- Mašek O, Budarin V, Gronnow M, et al. Microwave and slow pyrolysis biochar—Comparison of physical and functional properties. Journal of Analytical and Applied Pyrolysis. 2013; 100: 41-48. doi: 10.1016/j.jaap.2012.11.015
- Ahmad M, Rajapaksha AU, Lim JE, et al. Biochar as a sorbent for contaminant management in soil and water: A review. Chemosphere. 2014; 99: 19-33. doi: 10.1016/j.chemosphere.2013.10.071
- Tan X, Liu Y, Zeng G, et al. Application of biochar for the removal of pollutants from aqueous solutions. Chemosphere. 2015; 125: 70-85. doi: 10.1016/j.chemosphere.2014.12.058
- Manyà JJ, Azuara M, Manso JA. Biochar production through slow pyrolysis of different biomass materials: Seeking the best operating conditions. Biomass and Bioenergy. 2018; 117: 115-123. doi: 10.1016/j.biombioe.2018.07.019
- Escalante A, Pérez G, Hidalgo C, et al. Biocarbon (biochar) I: Nature, history, manufacture and use in soil Biocarbon (biochar) I: Nature, history, manufacture and use in soil. Terra Latinoamericana. 2016; 34(3): 367-382.
- Lehmann J. Bio-Energy in the Black. Frontiers in Ecology and the Environment. 2007; 5: 381-387. doi: 10.1890/1540- 9295(2007)5[381:BITB]2.0.CO;2
- Morseletto P. Targets for a circular economy. Resources, Conservation and Recycling. 2020; 153: 104553. doi: 10.1016/j.resconrec.2019.104553
- Sizmur T, Fresno T, Akgül G, et al. Biochar modification to enhance sorption of inorganics from water. Bioresource Technology. 2017; 246: 34-47. doi: 10.1016/j.biortech.2017.07.082
- Inyang M, Dickenson E. The potential role of biochar in the removal of organic and microbial contaminants from potable and reuse water: A review. Chemosphere. 2015; 134: 232-240. doi: 10.1016/j.chemosphere.2015.03.072
- He J, Strezov V, Kan T, et al. Slow pyrolysis of metal(loid)-rich biomass from phytoextraction: characterisation of biomass, biochar and bio-oil. Energy Procedia. 2019; 160: 178-185. doi: 10.1016/j.egypro.2019.02.134
- Campos P, Miller AZ, Knicker H, et al. Chemical, physical and morphological properties of biochars produced from agricultural residues: Implications for their use as soil amendment. Waste Management. 2020; 105: 256-267. doi: 10.1016/j.wasman.2020.02.013
- Kim WK, Shim T, Kim YS, et al. Characterization of cadmium removal from aqueous solution by biochar produced from a giant Miscanthus at different pyrolytic temperatures. Bioresource Technology. 2013; 138: 266-270. doi: 10.1016/j.biortech.2013.03.186
- Nguyen VT, Nguyen TB, Chen CW, et al. Influence of pyrolysis temperature on polycyclic aromatic hydrocarbons production and tetracycline adsorption behavior of biochar derived from spent coffee ground. Bioresource Technology. 2019; 284: 197-203. doi: 10.1016/j.biortech.2019.03.096
- Lee Y, Eum PRB, Ryu C, et al. Characteristics of biochar produced from slow pyrolysis of Geodae-Uksae 1. Bioresource Technology. 2013; 130: 345-350. doi: 10.1016/j.biortech.2012.12.012
- Arroyave-Manco JC, Arboleda-Echavarria JC, Hoyos-Ayala DA, et al. ZLTA and FAU eolites obtained from fly ash and their application in chromium removal (Spanish). DYNA. 2018; 85(204): 150-160. doi: 10.15446/dyna.v85n204.67096
- Zhang ZB, Cao XH, Liang P, et al. Adsorption of uranium from aqueous solution using biochar produced by hydrothermal carbonization. Journal of Radioanalytical and Nuclear Chemistry. 2013; 295(2): 1201-1208. doi: 10.1007/s10967-012-2017-2
- Abdel-Fattah TM, Mahmoud ME, Ahmed SB, et al. Biochar from woody biomass for removing metal contaminants and carbon sequestration. Journal of Industrial and Engineering Chemistry. 2015; 22: 103-109. doi: 10.1016/j.jiec.2014.06.030
- Xu X, Cao X, Zhao L. Comparison of rice husk- and dairy manure-derived biochars for simultaneously removing heavy metals from aqueous solutions: Role of mineral components in biochars. Chemosphere. 2013; 92(8): 955-961. doi: 10.1016/j.chemosphere.2013.03.009
- Zubair M, Manzar MS, Mu’azu ND, et al. Functionalized MgAl-layered hydroxide intercalated date-palm biochar for Enhanced Uptake of Cationic dye: Kinetics, isotherm and thermodynamic studies. Applied Clay Science. 2020; 190: 105587. doi: 10.1016/j.clay.2020.105587
- Vyavahare GD, Gurav RG, Jadhav PP, et al. Response surface methodology optimization for sorption of malachite green dye on sugarcane bagasse biochar and evaluating the residual dye for phyto and cytogenotoxicity. Chemosphere. 2018; 194: 306-315. doi: 10.1016/j.chemosphere.2017.11.180
- Wang Q, Wang B, Lee X, et al. Sorption and desorption of Pb(II) to biochar as affected by oxidation and pH. Science of The Total Environment. 2018; 634: 188-194. doi: 10.1016/j.scitotenv.2018.03.189
- Tran HN, Tomul F, Thi Hoang Ha N, et al. Innovative spherical biochar for pharmaceutical removal from water: Insight into adsorption mechanism. Journal of Hazardous Materials. 2020; 394: 122255. doi: 10.1016/j.jhazmat.2020.122255
- Shan R, Shi Y, Gu J, et al. Aqueous Cr(VI) removal by biochar derived from waste mangosteen shells: Role of pyrolysis and modification on its absorption process. Journal of Environmental Chemical Engineering. 2020; 8(4): 103885. doi: 10.1016/j.jece.2020.103885
- Qi F, Yan Y, Lamb D, et al. Thermal stability of biochar and its effects on cadmium sorption capacity. Bioresource Technology. 2017; 246: 48-56. doi: 10.1016/j.biortech.2017.07.033
- Sun L, Wan S, Luo W. Biochars prepared from anaerobic digestion residue, palm bark, and eucalyptus for adsorption of cationic methylene blue dye: Characterization, equilibrium, and kinetic studies. Bioresource Technology. 2013; 140: 406-413. doi: 10.1016/j.biortech.2013.04.116
- Idrees M, Batool S, Kalsoom T, et al. Animal manure-derived biochars produced via fast pyrolysis for the removal of divalent copper from aqueous media. Journal of Environmental Management. 2018; 213: 109-118. doi: 10.1016/j.jenvman.2018.02.003
- Lonappan L, Rouissi T, Brar SK, et al. Adsorption of diclofenac onto different biochar microparticles: Dataset —Characterization and dosage of biochar. Data in Brief. 2018; 16: 460-465. doi: 10.1016/j.dib.2017.10.041
- Gokulan R, Avinash A, Prabhu GG, et al. Remediation of remazol dyes by biochar derived from Caulerpa scalpelliformis—An eco-friendly approach. Journal of Environmental Chemical Engineering. 2019; 7(5): 103297. doi: 10.1016/j.jece.2019.103297
- Lu L, Lin Y, Chai Q, et al. Removal of acenaphthene by biochar and raw biomass with coexisting heavy metal and phenanthrene. Colloids and Surfaces A: Physicochemical and Engineering Aspects. 2018; 558: 103-109. doi: 10.1016/j.colsurfa.2018.08.057
- Deng Y, Huang S, Dong C, et al. Competitive adsorption behaviour and mechanisms of cadmium, nickel and ammonium from aqueous solution by fresh and ageing rice straw biochars. Bioresource Technology. 2020; 303: 122853. doi: 10.1016/j.biortech.2020.122853
- Ni BJ, Huang QS, Wang C, et al. Competitive adsorption of heavy metals in aqueous solution onto biochar derived from anaerobically digested sludge. Chemosphere. 2019; 219: 351-357. doi: 10.1016/j.chemosphere.2018.12.053
- Wang S, Ai S, Nzediegwu C, et al. Carboxyl and hydroxyl groups enhance ammonium adsorption capacity of iron (III) chloride and hydrochloric acid modified biochars. Bioresource Technology. 2020; 309: 123390. doi: 10.1016/j.biortech.2020.123390
- Liu J, Huang Z, Chen Z, et al. Resource utilization of swine sludge to prepare modified biochar adsorbent for the efficient removal of Pb(II) from water. Journal of Cleaner Production. 2020; 257: 120322. doi: 10.1016/j.jclepro.2020.120322
- Khan N, Chowdhary P, Ahmad A, et al. Hydrothermal liquefaction of rice husk and cow dung in Mixed-Bed-Rotating Pyrolyzer and application of biochar for dye removal. Bioresource Technology. 2020; 309: 123294. doi: 10.1016/j.biortech.2020.123294
- Han Y, Cao X, Ouyang X, et al. Adsorption kinetics of magnetic biochar derived from peanut hull on removal of Cr (VI) from aqueous solution: Effects of production conditions and particle size. Chemosphere. 2016; 145: 336-341. doi: 10.1016/j.chemosphere.2015.11.050
- Veiga PA da S, Schultz J, Matos TT da S, et al. Production of high-performance biochar using a simple and low-cost method: Optimization of pyrolysis parameters and evaluation for water treatment. Journal of Analytical and Applied Pyrolysis. 2020; 148: 104823. doi: 10.1016/j.jaap.2020.104823
- Harikishore Kumar Reddy D, Vijayaraghavan K, Kim JA, et al. Valorisation of post-sorption materials: Opportunities, strategies, and challenges. Advances in Colloid and Interface Science. 2017; 242: 35-58. doi: 10.1016/j.cis.2016.12.002
- Bhomick PC, Supong A, Baruah M, et al. Pine Cone biomass as an efficient precursor for the synthesis of activated biocarbon for adsorption of anionic dye from aqueous solution: Isotherm, kinetic, thermodynamic and regeneration studies. Sustainable Chemistry and Pharmacy. 2018; 10: 41-49. doi: 10.1016/j.scp.2018.09.001
- Essandoh M, Kunwar B, Pittman CU, et al. Sorptive removal of salicylic acid and ibuprofen from aqueous solutions using pine wood fast pyrolysis biochar. Chemical Engineering Journal. 2015; 265: 219-227. doi: 10.1016/j.cej.2014.12.006
- Bădescu IS, Bulgariu D, Ahmad I, et al. Valorisation possibilities of exhausted biosorbents loaded with metal ions—A review. Journal of Environmental Management. 2018; 224: 288-297. doi: 10.1016/j.jenvman.2018.07.066
- Truong HB, Ike IA, Ok YS, et al. Polyethyleneimine modification of activated fly ash and biochar for enhanced removal of natural organic matter from water via adsorption. Chemosphere. 2020; 243: 125454. doi: 10.1016/j.chemosphere.2019.125454
- Zhang Y, Yue X, Xu W, et al. Amino modification of rice straw-derived biochar for enhancing its cadmium (II) ions adsorption from water. Journal of Hazardous Materials. 2019; 379: 120783. doi: 10.1016/j.jhazmat.2019.120783
- Oginni O, Yakaboylu GA, Singh K, et al. Phosphorus adsorption behaviors of MgO modified biochars derived from waste woody biomass resources. Journal of Environmental Chemical Engineering. 2020; 8(2): 103723. doi: 10.1016/j.jece.2020.103723
- Li H, Dong X, da Silva EB, et al. Mechanisms of metal sorption by biochars: Biochar characteristics and modifications. Chemosphere. 2017; 178: 466-478. doi: 10.1016/j.chemosphere.2017.03.072
- Zhou X, Zhou J, Liu Y, et al. Preparation of iminodiacetic acid-modified magnetic biochar by carbonization, magnetization and functional modification for Cd(II) removal in water. Fuel. 2018; 233: 469-479. doi: 10.1016/j.fuel.2018.06.075
- Feng Y, Liu P, Wang Y, et al. Mechanistic investigation of mercury removal by unmodified and Fe-modified biochars based on synchrotron-based methods. Science of The Total Environment. 2020; 719: 137435. doi: 10.1016/j.scitotenv.2020.137435
Refbacks
- There are currently no refbacks.
Copyright (c) 2024 Deiby Présiga-López, Ainhoa Rubio-Clemente, Juan F. Pérez

This work is licensed under a Creative Commons Attribution 4.0 International License.
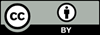
This site is licensed under a Creative Commons Attribution 4.0 International License (CC BY 4.0).
.jpg)
Beijing University of Technology, China