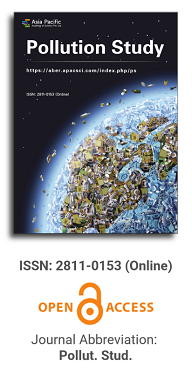
Asia Pacific Academy of Science Pte. Ltd. (APACSCI) specializes in international journal publishing. APACSCI adopts the open access publishing model and provides an important communication bridge for academic groups whose interest fields include engineering, technology, medicine, computer, mathematics, agriculture and forestry, and environment.
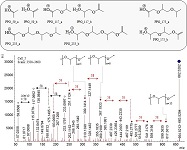
The (partial) replacement of synthetic polymers with bioplastics is due to increased production of conventional packaging plastics causing for severe environmental pollution with plastics waste. The bioplastics, however, represent complex mixtures of known and unknown (bio)polymers, fillers, plasticizers, stabilizers, flame retardant, pigments, antioxidants, hydrophobic polymers such as poly(lactic acid), polyethylene, polyesters, glycol, or poly(butylene succinate), and little is known of their chemical safety for both the environment and the human health. Polymerization reactions of bioplastics can produce no intentionally added chemicals to the bulk material, which could be toxic, as well. When polymers are used to food packing, then the latter chemicals could also migrate from the polymer to food. This fact compromises the safety for consumers, as well. The scarce data on chemical safety of bioplastics makes a gap in knowledge of their toxicity to humans and environment. Thus, development of exact analytical protocols for determining chemicals of bioplastics in environmental and food samples as well as packing polymers can only provide warrant for reliable conclusive evidence of their safety for both the human health and the environment. The task is compulsory according to legislation Directives valid to environmental protection, food control, and assessment of the risk to human health. The quantitative and structural determination of analytes is primary research task of analysis of polymers. The methods of mass spectrometry are fruitfully used for these purposes. Methodological development of exact analytical mass spectrometric tools for reliable structural analysis of bioplastics only guarantees their safety, efficacy, and quality to both humans and environment. This study, first, highlights innovative stochastic dynamics equations processing exactly mass spectrometric measurands and, thus, producing exact analyte quantification and 3D molecular and electronic structural analyses. There are determined synthetic polymers such as poly(ethylenglycol), poly(propylene glycol), and polyisoprene as well as biopolymers in bags for foodstuffs made from renewable cellulose and starch, and containing, in total within the 20,416–17,495 chemicals per sample of the composite biopolymers. Advantages of complementary employment in mass spectrometric methods and Fourier transform infrared spectroscopy is highlighted. The study utilizes ultra-high resolution electrospray ionization mass spectrometric and Fourier transform infrared spectroscopic data on biodegradable plastics bags for foodstuffs; high accuracy quantum chemical static methods, molecular dynamics; and chemometrics. There is achieved method performance |r| = 0.99981 determining poly(propylene glycol) in bag for foodstuff containing 20,416 species and using stochastic dynamics mass spectrometric formulas. The results highlight their great capability and applicability to the analytical science as well as relevance to both the fundamental research and to the industry.
The advancement and future outlook of bioremediation techniques for locations contaminated with petroleum and petrochemicals
Vol 3, Issue 1, 2022
Issue release: 31 December 2022
VIEWS - 7520 (Abstract)
Download PDF
Abstract
The exploitation of petroleum assets fuels economic and societal progress, but it also leads to extensive contamination of land with oil and petrochemicals, which increasingly threatens the environmental integrity of these areas. Bioremediation has emerged as a favored approach for addressing this issue due to its environmentally benign nature and absence of secondary pollution. This discourse encapsulates the latest advancements in bioremediation strategies, particularly those involving microbial and phytoremediation techniques for tackling petroleum and petrochemical contamination. It also examines the constraints of current bioremediation practices and envisions future research directions. The objective is to enhance the efficacy of bioremediation for contaminated sites and to establish a scientific foundation that will support the evolution and comprehensive application of bioremediation technologies.
Keywords
References
1. Zhou Q, Song Y. Principles and methods of contaminated soil remediation. Beijing: Science Press; 2004.
2. Sun J, Pan L, Zhan Y, et al. Contamination of phthalate esters, organochlorine pesticides and polybrominated diphenyl ethers in agricultural soils from the Yangtze River Delta of China. Science of The Total Environment. 2016; 544: 670-676. doi: 10.1016/j.scitotenv.2015.12.012
3. Zhong Y, Zhu L. Distribution, input pathway and soil-air exchange of polycyclic aromatic hydrocarbons in Banshan Industry Park, China. Science of The Total Environment. 2013; 444: 177-182. doi: 10.1016/j.scitotenv.2012.11.091
4. Anac, Jordi D, Claudia M, et al. Factors influencing the soil-air partitioning and the strength of soils as a secondary source of polychlorinated biphenyls to the atmosphere. Environmental Science & Technology. 2011; 45(11): 4785-4792. doi: 10.1021/es200400e
5. Liu Y, Li S, Ni Z, et al. Pesticides in persimmons, jujubes and soil from China: Residue levels, risk assessment and relationship between fruits and soils. Science of The Total Environment. 2016; 542: 620-628. doi: 10.1016/j.scitotenv.2015.10.148.
6. Silva R, Almeida D, Rufino R, et al. Applications of Biosurfactants in the Petroleum Industry and the Remediation of Oil Spills. International Journal of Molecular Sciences. 2014; 15(7): 12523-12542. doi: 10.3390/ijms150712523
7. Bidja Abena MT, Li T, Shah MN, et al. Biodegradation of total petroleum hydrocarbons (TPH) in highly contaminated soils by natural attenuation and bioaugmentation. Chemosphere. 2019; 234: 864-874. doi: 10.1016/j.chemosphere.2019.06.111
8. Eweje G. Environmental Costs and Responsibilities Resulting from Oil Exploitation in Developing Countries: The Case of the Niger Delta of Nigeria. Journal of Business Ethics. 2006; 69(1): 27-56. doi: 10.1007/s10551-006-9067-8
9. Abioye OP, Agamuthu P, Abdul Aziz AR. Phytotreatment of soil contaminated with used lubricating oil using Hibiscus cannabinus. Biodegradation. 2011; 23(2): 277-286. doi: 10.1007/s10532-011-9506-9
10. Roy AS, Baruah R, Borah M, et al. Bioremediation potential of native hydrocarbon degrading bacterial strains in crude oil contaminated soil under microcosm study. International Biodeterioration & Biodegradation. 2014; 94: 79-89. doi: 10.1016/j.ibiod.2014.03.024
11. Sherwood MK, Cassidy DP. Modified Fenton oxidation of diesel fuel in arctic soils rich in organic matter and iron. Chemosphere. 2014; 113: 56-61. doi: 10.1016/j.chemosphere.2014.04.048
12. Gan S, Lau EV, Ng HK. Remediation of soils contaminated with polycyclic aromatic hydrocarbons (PAHs). Journal of Hazardous Materials. 2009; 172(2-3): 532-549. doi: 10.1016/j.jhazmat.2009.07.118
13. Pazos M, Plaza A, Martín M, et al. The impact of electrokinetic treatment on a loamy-sand soil properties. Chemical Engineering Journal. 2012; 183: 231-237. doi: 10.1016/j.cej.2011.12.067
14. Anthony EJ, Wang J. Pilot plant investigations of thermal remediation of tar-contaminated soil and oil-contaminated gravel. Fuel. 2006; 85(4): 443-450. doi: 10.1016/j.fuel.2005.08.012
15. Falciglia PP, Giustra MG, Vagliasindi FGA. Low-temperature thermal desorption of diesel polluted soil: Influence of temperature and soil texture on contaminant removal kinetics. Journal of Hazardous Materials. 2011; 185(1): 392-400. doi: 10.1016/j.jhazmat.2010.09.046
16. Kavitha V, Mandal AB, Gnanamani A. Microbial biosurfactant mediated removal and/or solubilization of crude oil contamination from soil and aqueous phase: An approach with Bacillus licheniformis MTCC 5514. International Biodeterioration & Biodegradation. 2014; 94: 24-30. doi: 10.1016/j.ibiod.2014.04.028
17. Malina G, Grotenhuis JTC, Rulkens WH. Vapor Extraction/Bioventing Sequential Treatment of Soil Contaminated with Volatile and SemiVolatile Hydrocarbon Mixtures. Bioremediation Journal. 2002; 6(2): 159-176. doi: 10.1080/10588330208951211
18. Wang X, Cai Z, Zhou Q, et al. Bioelectrochemical stimulation of petroleum hydrocarbon degradation in saline soil using U‐tube microbial fuel cells. Biotechnology and Bioengineering. 2011; 109(2): 426-433. doi: 10.1002/bit.23351
19. Gielnik A, Pechaud Y, Huguenot D, et al. Effect of digestate application on microbial respiration and bacterial communities’ diversity during bioremediation of weathered petroleum hydrocarbons contaminated soils. Science of The Total Environment. 2019; 670: 271-281. doi: 10.1016/j.scitotenv.2019.03.176
20. Hoff RZ. Bioremediation: An overview of its development and use for oil spill cleanup. Marine Pollution Bulletin. 1993; 26(9): 476-481.
21. Mancera-López ME, Esparza-García F, Chávez-Gómez B, et al. Bioremediation of an aged hydrocarbon-contaminated soil by a combined system of biostimulation–bioaugmentation with filamentous fungi. International Biodeterioration & Biodegradation. 2008; 61(2): 151-160. doi: 10.1016/j.ibiod.2007.05.012
22. Potin O, Rafin C, Veignie E. Bioremediation of an aged polycyclic aromatic hydrocarbons (PAHs)-contaminated soil by filamentous fungi isolated from the soil. International Biodeterioration & Biodegradation. 2004; 54(1): 45-52. doi: 10.1016/j.ibiod.2004.01.003
23. Zhang T, Huang Y, Ji L, et al. Research progress of bioremediation technology for oil contaminated soil (Chinese). Shandong science. 2020; 33(5): 106-112,126.
24. Varjani SJ, Rana DP, Jain AK, et al. Synergistic ex-situ biodegradation of crude oil by halotolerant bacterial consortium of indigenous strains isolated from on shore sites of Gujarat, India. International Biodeterioration & Biodegradation. 2015; 103: 116-124. doi: 10.1016/j.ibiod.2015.03.030
25. Covino S, D’Annibale A, Stazi SR, et al. Assessment of degradation potential of aliphatic hydrocarbons by autochthonous filamentous fungi from a historically polluted clay soil. Science of The Total Environment. 2015; 505: 545-554. doi: 10.1016/j.scitotenv.2014.10.027
26. Ramadass K, Megharaj M, Venkateswarlu K, et al. Bioavailability of weathered hydrocarbons in engine oil-contaminated soil: Impact of bioaugmentation mediated by Pseudomonas spp. on bioremediation. Science of The Total Environment. 2018; 636: 968-974. doi: 10.1016/j.scitotenv.2018.04.379
27. Qu Y, Ma J, Zhong J, et al. Study on microbial synergistic degradation of deep oil contaminated soil. Oil and gas field environmental protection. 2016; 26(5): 6-9,17.
28. Wu G, Li X, Coulon F, et al. Recycling of solvent used in a solvent extraction of petroleum hydrocarbons contaminated soil. Journal of Hazardous Materials. 2011; 186(1): 533-539. doi: 10.1016/j.jhazmat.2010.11.041
29. Wu G, Zhu X, Ji H, et al. Molecular modeling of interactions between heavy crude oil and the soil organic matter coated quartz surface. Chemosphere. 2015; 119: 242-249. doi: 10.1016/j.chemosphere.2014.06.030
30. Yan G, Ma W, Chen C, et al. Combinations of Surfactant Flushing and Bioremediation for Removing Fuel Hydrocarbons from Contaminated Soils. CLEAN – Soil, Air, Water. 2016; 44(8): 984-991. doi: 10.1002/clen.201500571
31. Lu M, Zhang Z, Qiao W, et al. Remediation of petroleum-contaminated soil after composting by sequential treatment with Fenton-like oxidation and biodegradation. Bioresource Technology. 2010; 101(7): 2106-2113. doi: 10.1016/j.biortech.2009.11.002
32. Kim I, Lee M. Pilot scale feasibility study for in-situ chemical oxidation using H2O2 solution conjugated with biodegradation to remediate a diesel contaminated site. Journal of Hazardous Materials. 2012; 241-242: 173-181. doi: 10.1016/j.jhazmat.2012.09.022
33. Gong XB. Remediation of weathered petroleum oil-contaminated soil using a combination of biostimulation and modified Fenton oxidation. International Biodeterioration & Biodegradation. 2012; 70: 89-95. doi: 10.1016/j.ibiod.2012.02.004
34. Lahel A, Fanta AB, Sergienko N, et al. Effect of process parameters on the bioremediation of diesel contaminated soil by mixed microbial consortia. International Biodeterioration & Biodegradation. 2016; 113: 375-385. doi: 10.1016/j.ibiod.2016.05.005
35. Wang C, Zhang Z, Xu W, et al. Electrokinetic-Assisted Bioremediation of Field Soil with Historic Polycyclic Aromatic Hydrocarbon Contamination. Environmental Engineering Science. 2016; 33(1): 44-52. doi: 10.1089/ees.2014.0286
36. Prakash AA, Prabhu NS, Rajasekar A, et al. Bio-electrokinetic remediation of crude oil contaminated soil enhanced by bacterial biosurfactant. Journal of Hazardous Materials. 2021; 405: 124061. doi: 10.1016/j.jhazmat.2020.124061
37. Wang H, Luo H, Fallgren PH, et al. Bioelectrochemical system platform for sustainable environmental remediation and energy generation. Biotechnology Advances. 2015; 33(3-4): 317-334. doi: 10.1016/j.biotechadv.2015.04.003
38. Wang X, Feng C, Ding N, et al. Accelerated OH– Transport in Activated Carbon Air Cathode by Modification of Quaternary Ammonium for Microbial Fuel Cells. Environmental Science & Technology. 2014; 48(7): 4191-4198. doi: 10.1021/es5002506
39. Lu L, Yazdi H, Jin S, et al. Enhanced bioremediation of hydrocarbon-contaminated soil using pilot-scale bioelectrochemical systems. Journal of Hazardous Materials. 2014; 274: 8-15. doi: 10.1016/j.jhazmat.2014.03.060
40. Zhou Q, Liu J, Xue S, et al. Remediation practices and cases of polluted environment (Chinese). Beijing: Chemical Industry Press; 2021.
41. Ebadi A, Khoshkholgh Sima NA, Olamaee M, et al. Remediation of saline soils contaminated with crude oil using the halophyte Salicornia persica in conjunction with hydrocarbon-degrading bacteria. Journal of Environmental Management. 2018; 219: 260-268. doi: 10.1016/j.jenvman.2018.04.115
42. Kotoky R, Pandey P. Rhizosphere mediated biodegradation of benzo(A)pyrene by surfactin producing soil bacilli applied through Melia azedarach rhizosphere. International Journal of Phytoremediation. 2019; 22(4): 363-372. doi: 10.1080/15226514.2019.1663486
43. Xia M, Chakraborty R, Terry N, et al. Promotion of saltgrass growth in a saline petroleum hydrocarbons contaminated soil using a plant growth promoting bacterial consortium. International Biodeterioration & Biodegradation. 2020; 146: 104808. doi: 10.1016/j.ibiod.2019.104808
44. Cheng L, Wang Y, Cai Z, et al. Phytoremediation of petroleum hydrocarbon-contaminated saline-alkali soil by wild ornamental Iridaceae species. International Journal of Phytoremediation. 2016; 19(3): 300-308. doi: 10.1080/15226514.2016.1225282
45. Zhou Q, Tang J, Wei S. Geochemical basis and related theories of environmental green restoration. Journal of ecology and rural environment. 2020; 36(1): 1-10.
46. Brereton NJB, Gonzalez E, Desjardins D, et al. Co-cropping with three phytoremediation crops influences rhizosphere microbiome community in contaminated soil. Science of The Total Environment. 2020; 711: 135067. doi: 10.1016/j.scitotenv.2019.135067
47. Dacunha ACB, Sabedot S, Sampaio CH, et al. Salix rubens and Salix triandra Species as Phytoremediators of Soil Contaminated with Petroleum-Derived Hydrocarbons. Water, Air, & Soil Pollution. 2012; 223(8): 4723-4731. doi: 10.1007/s11270-012-1228-z
48. Desjardins D, Brereton NJB, Marchand L, et al. Complementarity of three distinctive phytoremediation crops for multiple-trace element contaminated soil. Science of The Total Environment. 2018; 610-611: 1428-1438. doi: 10.1016/j.scitotenv.2017.08.196
49. Miao X, Zhou Q. Research Progress on Influencing Factors of phytoremediation efficiency of contaminated soil. Journal of ecology. 2015; 34(3): 870-877.
50. Gu P, Zhou Q, Wang X, et al Study on remediation of contaminated soil by a benzo [a]. pyrene degrading bacterium mirabilis jalapa. Journal of environmental science. 2018; 38(4): 1613-1620.
51. Shang Q, Zhang X, Li Z, et al. Synergistic remediation of oil contaminated soil by straw and microorganism. Journal of petroleum. 2018; 34(6): 1203-1210.
52. Tang J, Dong J, Niu X, et al. A method for remediation of oil contaminated saline alkali soil with ryegrass and high-efficiency microorganisms. Springer, 2010; 34: 2010102294162.
53. Hou J, Liu W, Wang B, et al. PGPR enhanced phytoremediation of petroleum contaminated soil and rhizosphere microbial community response. Chemosphere. 2015; 138: 592-598. doi: 10.1016/j.chemosphere.2015.07.025
54. Wang MC, Chen YT, Chen SH, et al. Phytoremediation of pyrene contaminated soils amended with compost and planted with ryegrass and alfalfa. Chemosphere. 2012; 87(3): 217-225. doi: 10.1016/j.chemosphere.2011.12.063
55. Sun Y, Xu Y, Zhou Q, et al. The potential of gibberellic acid 3 (GA3) and Tween-80 induced phytoremediation of co-contamination of Cd and Benzo[a]pyrene (B[a]P) using Tagetes patula. Journal of Environmental Management. 2013; 114: 202-208. doi: 10.1016/j.jenvman.2012.09.018
56. Rostami S, Azhdarpoor A, Rostami M, et al. The effects of simultaneous application of plant growth regulators and bioaugmentation on improvement of phytoremediation of pyrene contaminated soils. Chemosphere. 2016; 161: 219-223. doi: 10.1016/j.chemosphere.2016.07.026
57. Agnello AC, Huguenot D, Van Hullebusch ED, et al. Enhanced Phytoremediation: A Review of Low Molecular Weight Organic Acids and Surfactants Used as Amendments. Critical Reviews in Environmental Science and Technology. 2014; 44(22): 2531-2576. doi: 10.1080/10643389.2013.829764
58. Firdaus EB. Chelate assisted phytoextraction using oilseed brassicas. Dordrecht: Springer Netherlands; 2012. pp. 289-311.
59. Fadhile Almansoory A, Abu Hasan H, Idris M, et al. Potential application of a biosurfactant in phytoremediation technology for treatment of gasoline-contaminated soil. Ecological Engineering. 2015; 84: 113-120. doi: 10.1016/j.ecoleng.2015.08.001
60. Yue Z, Zhou Q. Application and Prospect of nano materials in remediation of organic contaminated soil. Journal of Agricultural Environmental Sciences. 2017; 36(10): 1929-1937.
61. Zhou Q, Ma S, Zhan S. Superior photocatalytic disinfection effect of Ag-3D ordered mesoporous CeO2 under visible light. Applied Catalysis B: Environmental. 2018; 224: 27-37. doi: 10.1016/j.apcatb.2017.10.032
62. Hu X, Zhou Q. Health and Ecosystem Risks of Graphene. Chemical Reviews. 2013; 113(5): 3815-3835. doi: 10.1021/cr300045n
63. Gao YY, Zhou QX. Application of Nanoscale Zero Valent Iron Combined with Impatiens Balsamina to Remediation of E-Waste Contaminated Soils. Advanced Materials Research. 2013; 790: 73-76. doi: 10.4028/www.scientific.net/amr.790.73
64. Gao Y, Zhou Q. Application and Prospect of nano zero valent iron in remediation of contaminated soil. Journal of Agricultural Environmental Sciences. 2013; 32(3): 418-425.
65. Song B, Xu P, Chen M, et al. Using nanomaterials to facilitate the phytoremediation of contaminated soil. Critical Reviews in Environmental Science and Technology. 2019; 49(9): 791-824. doi: 10.1080/10643389.2018.1558891
66. Kolton M, Graber ER, Tsehansky L, et al. Biochar‐stimulated plant performance is strongly linked to microbial diversity and metabolic potential in the rhizosphere. New Phytologist. 2016; 213(3): 1393-1404. doi: 10.1111/nph.14253
67. Cao Y, Yang B, Song Z, et al. Wheat straw biochar amendments on the removal of polycyclic aromatic hydrocarbons (PAHs) in contaminated soil. Ecotoxicology and Environmental Safety. 2016; 130: 248-255. doi: 10.1016/j.ecoenv.2016.04.033
68. Cang L, Wang Q, Zhou D, et al. Effects of electrokinetic-assisted phytoremediation of a multiple-metal contaminated soil on soil metal bioavailability and uptake by Indian mustard. Separation and Purification Technology. 2011; 79(2): 246-253. doi: 10.1016/j.seppur.2011.02.016
69. Acosta-Santoyo G, Cameselle C, Bustos E. Electrokinetic – Enhanced ryegrass cultures in soils polluted with organic and inorganic compounds. Environmental Research. 2017; 158: 118-125. doi: 10.1016/j.envres.2017.06.004
70. Rocha IMV, Silva KNO, Silva DR, et al. Coupling electrokinetic remediation with phytoremediation for depolluting soil with petroleum and the use of electrochemical technologies for treating the effluent generated. Separation and Purification Technology. 2019; 208: 194-200. doi: 10.1016/j.seppur.2018.03.012
71. Cameselle C, Chirakkara RA, Reddy KR. Electrokinetic-enhanced phytoremediation of soils: Status and opportunities. Chemosphere. 2013; 93(4): 626-636. doi: 10.1016/j.chemosphere.2013.06.029
Refbacks
- There are currently no refbacks.
Copyright (c) 2022 Qixing Zhou, Haiyin Zhan

This work is licensed under a Creative Commons Attribution 4.0 International License.
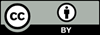
This site is licensed under a Creative Commons Attribution 4.0 International License (CC BY 4.0).
.jpg)
Prof. Hongxing Dai
Beijing University of Technology, China