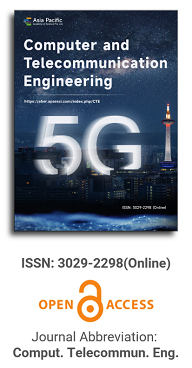
Design of magneto-inductive waveguide in 2-d magnetic metamaterial structure for wireless power transfer and near-field communications
Vol 2, Issue 4, 2024
Download PDF
Abstract
Recently, significant research has been conducted on magnetic metamaterials that exhibit negative permeability and operate within the GHz and MHz frequency ranges. These metamaterial structures can be utilized to improve the efficiency of near-field wireless power transfer systems, subterranean communication, and position sensors. However, in most cases, they are only designed to work for a single application. This study focuses on examining the transmission of magneto-inductive waves in magnetic metamaterial structures with ordered arrangements. This structure can be used simultaneously for wireless power transfer and near-field communications. The unit cell is formed by a spiral with five turns that is implanted on a FR-4 substrate. An external capacitor was used to regulate the resonant frequency of the magnetic metamaterial unit cell. The properties of magneto-inductive waves, including reflection, transmission response, and field distribution on the waveguide, have been extensively computed and simulated. The obtained results indicate that both 1-dimensional and 2-dimensional magnetic metamaterial configurations possess the ability to conduct electromagnetic waves and propagate magnetic field energy at a frequency of 13.56 MHz. The straight and cross path configurations were also investigated to identify the optimal configuration on the 2-dimensional metamaterial slab.
Keywords
References
- Veselago VG. The Electrodynamics of Substances with Simultaneously Negative Values oF $epsilon$ and μ. Soviet Physics Uspekhi. 1968; 10(4): 509-514. doi: 10.1070/pu1968v010n04abeh003699
- Pendry JB, Holden AJ, Robbins DJ, et al. Magnetism from conductors and enhanced nonlinear phenomena. IEEE Transactions on Microwave Theory and Techniques. 1999; 47(11): 2075-2084. doi: 10.1109/22.798002
- Pendry JB, Holden AJ, Stewart WJ, et al. Extremely Low Frequency Plasmons in Metallic Mesostructures. Physical Review Letters. 1996; 76(25): 4773-4776. doi: 10.1103/physrevlett.76.4773
- Marqués R, Martel J, Mesa F, et al. Left-Handed-Media Simulation and Transmission of EM Waves in Subwavelength Split-Ring-Resonator-Loaded Metallic Waveguides. Physical Review Letters. 2002; 89(18). doi: 10.1103/physrevlett.89.183901
- Zheng H, Pham TS, Chen L, et al. Metamaterial Perfect Absorbers for Controlling Bandwidth: Single-Peak/Multiple-Peaks/Tailored-Band/Broadband. Crystals. 2023; 14(1): 19. doi: 10.3390/cryst14010019
- Pendry J. Negative refraction. Contemporary Physics. 2004; 45(3): 191-202. doi: 10.1080/00107510410001667434
- Abdulkarim YI, Mohanty A, Acharya OP, et al. A Review on Metamaterial Absorbers: Microwave to Optical. Frontiers in Physics. 2022; 10. doi: 10.3389/fphy.2022.893791
- Almoneef TS, Ramahi OM. Metamaterial electromagnetic energy harvester with near unity efficiency. Applied Physics Letters. 2015; 106(15). doi: 10.1063/1.4916232
- Góra P, Łopato P. Metamaterials’ Application in Sustainable Technologies and an Introduction to Their Influence on Energy Harvesting Devices. Applied Sciences. 2023; 13(13): 7742. doi: 10.3390/app13137742
- Nguyen TT, Lim S. Design of Metamaterial Absorber using Eight-Resistive-Arm Cell for Simultaneous Broadband and Wide-Incidence-Angle Absorption. Scientific Reports. 2018; 8(1). doi: 10.1038/s41598-018-25074-8
- Pham TS, Zheng H, Chen L, et al. Wide-incident-angle, polarization-independent broadband-absorption metastructure without external resistive elements by using a trapezoidal structure. Scientific Reports. 2024; 14(1). doi: 10.1038/s41598-024-60171-x
- Singh G, Ni R, Marwaha A. A Review of Metamaterials and its Applications. International Journal of Engineering Trends and Technology. 2015; 19(6): 305-310. doi: 10.14445/22315381/ijett-v19p254
- Adepoju W, Bhattacharya I, Sanyaolu M, et al. Critical Review of Recent Advancement in Metamaterial Design for Wireless Power Transfer. IEEE Access. 2022; 10: 42699-42726. doi: 10.1109/access.2022.3167443
- Rong C, Yan L, Li L, et al. A Review of Metamaterials in Wireless Power Transfer. Materials. 2023; 16(17): 6008. doi: 10.3390/ma16176008
- Liao S, Qiao Z, Sui J, et al. Multifunctional Device for Circular to Linear Polarization Conversion and Absorption. Annalen der Physik. 2023; 535(7). doi: 10.1002/andp.202300195
- Liao S, Sui J, Zhang H. Switchable ultra-broadband absorption and polarization conversion metastructure controlled by light. Optics Express. 2022; 30(19): 34172. doi: 10.1364/oe.472336
- Guo Z, Gao C, Zhang H. Direction‐Dependent Janus Metasurface Supported by Waveguide Structure with Spoof Surface Plasmon Polariton Modes. Advanced Materials Technologies. 2022; 8(2). doi: 10.1002/admt.202200435
- Guo S, Hu C, Zhang H. Unidirectional ultrabroadband and wide-angle absorption in graphene-embedded photonic crystals with the cascading structure comprising the Octonacci sequence. Journal of the Optical Society of America B. 2020; 37(9): 2678. doi: 10.1364/josab.399048
- Anwar S, Khan M. Simple design of metamaterial sensor for biomedical sensing. Physica Scripta. 2022; 97(12): 125504. doi: 10.1088/1402-4896/ac9a87
- Fan J, Zhang L, Wei S, et al. A review of additive manufacturing of metamaterials and developing trends. Materials Today. 2021; 50: 303-328. doi: 10.1016/j.mattod.2021.04.019
- Lu C, Huang X, Tao X, et al. Comprehensive Analysis of Side-Placed Metamaterials in Wireless Power Transfer System. IEEE Access. 2020; 8: 152900-152908. doi: 10.1109/access.2020.3017492
- Hong Hiep LT, Pham TS, Khuyen BX, et al. Enhanced transmission efficiency of magneto-inductive wave propagating in non-homogeneous 2-D magnetic metamaterial array. Physica Scripta. 2022; 97(2): 025504. doi: 10.1088/1402-4896/ac4a3a
- Hiep LTH, Khuyen BX, Tung BS, et al. Flexible Magnetic Metasurface with Defect Cavity for Wireless Power Transfer System. Materials. 2022; 15(19): 6583. doi: 10.3390/ma15196583
- Wu Z, Yu H, Schreurs D, et al. Wireless power transfer based on 2D routing. Scientific Reports. 2022; 12(1). doi: 10.1038/s41598-022-22319-5
- Zeng Y, Lu C, Liu R, et al. Wireless Power and Data Transfer System Using Multidirectional Magnetic Coupler for Swarm AUVs. IEEE Transactions on Power Electronics. 2023; 38(2): 1440-1444. doi: 10.1109/tpel.2022.3214318
- Shamonina E, Kalinin VA, Ringhofer KH, et al. Magneto-inductive waveguide. Electronics Letters. 2002; 38(8): 371-373; doi: 10.1049/el:20020258
- Shamonina E, Solymar L. Properties of magnetically coupled metamaterial elements. Journal of Magnetism and Magnetic Materials. 2006; 300(1): 38-43. doi: 10.1016/j.jmmm.2005.10.028
- Liu W, Chau KT, Tian X, et al. Smart wireless power transfer—opportunities and challenges. Renewable and Sustainable Energy Reviews. 2023; 180: 113298. doi: 10.1016/j.rser.2023.113298
- Bennia F, Boudouda A, Nafa F. Optimal design of wireless power transfer coils for biomedical implants using machine learning and meta-heuristic algorithms. Electrical Engineering. 2024. doi: 10.1007/s00202-024-02345-4
- Celentano A, Paolino C, Pareschi F, et al. Mutual Inductance Measurement in Wireless Power Transfer Systems Operating in the MHz Range. IEEE Transactions on Circuits and Systems II: Express Briefs. 2024; 71(3): 1715-1720. doi: 10.1109/tcsii.2023.3334432
- Hoang MK, Xuan TK, Xuan TP, et al. A Design of Magnetic Resonant Wireless Power Transfer System using Flexible Resonator Coils. Journal of Magnetics. 2023; 28(3): 323-330. doi: 10.4283/jmag.2023.28.3.323
- Shah IA, Zada M, Shah SAA, et al. Flexible Metasurface-Coupled Efficient Wireless Power Transfer System for Implantable Devices. IEEE Transactions on Microwave Theory and Techniques. 2024; 72(4): 2534-2547. doi: 10.1109/tmtt.2023.3319050
- Jo S, Lee W, Lee H. Metasurface Patch for Wireless Power Transfer in Implantable Devices. Advanced Functional Materials. 2023; 33(38). doi: 10.1002/adfm.202300027
- Feis J, Solymar L, Shamonina E. Topological wireless communication in the stopband of magnetoinductive lines. Journal of Applied Physics. 2023; 133(22). doi: 10.1063/5.0146831
- Voronov A, Sydoruk O, Syms RRA. Power waves and scattering parameters in magneto-inductive systems. AIP Advances. 2021; 11(4). doi: 10.1063/5.0049806
- Pham TS, Khuyen BX, Tung BS, et al. Enhanced Efficiency of Asymmetric Wireless Power Transmission Using Defects in 2D Magnetic Metamaterials. Journal of Electronic Materials. 2020; 50(2): 443-449. doi: 10.1007/s11664-020-08586-w
- Choi J, Seo C. High-Efficiency Wireless Energy Transmission Using Magnetic Resonance Based on Negative Refractive Index Metamaterial. Progress In Electromagnetics Research. 2010; 106: 33-47. doi: 10.2528/pier10050609
- Hiep LTH, Bui HN, Tung BS, et al. Enhanced efficiency of magnetic resonant wireless power transfer system using rollable and foldable metasurface based on polyimide substrate. Applied Physics A. 2024; 130(7). doi: 10.1007/s00339-024-07684-4
- Zhi Sun, Akyildiz IF. Magnetic Induction Communications for Wireless Underground Sensor Networks. IEEE Transactions on Antennas and Propagation. 2010; 58(7): 2426-2435. doi: 10.1109/tap.2010.2048858
- Ranaweera ALAK, Duong TP, Lee JW. Experimental investigation of compact metamaterial for high efficiency mid-range wireless power transfer applications. Journal of Applied Physics. 2014; 116(4). doi: 10.1063/1.4891715
- Aydin K, Ozbay E. Capacitor-loaded split ring resonators as tunable metamaterial components. Journal of Applied Physics. 2007; 101(2). doi: 10.1063/1.2427110
- Pham TS, Bui HN, Lee JW. Wave propagation control and switching for wireless power transfer using tunable 2-D magnetic metamaterials. Journal of Magnetism and Magnetic Materials. 2019; 485: 126-135. doi: 10.1016/j.jmmm.2019.04.034
Refbacks
- There are currently no refbacks.
Copyright (c) 2024 Xuan Thanh Pham, Xuan Hung Nguyen, Kim Hoan Vu, Dinh Linh Trinh, Thanh Son Pham
License URL: https://creativecommons.org/licenses/by/4.0/
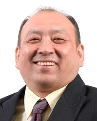
Prof. Maode Ma
Qatar University, Qatar
-
-
-
-
-
-
-
-
- J-Gate
-
-
-
-
-
-
The field of computer and telecommunications engineering is rapidly advancing, with the following being some of the latest developments.
more
We are pleased to congratulate the first anniversiry of the journal of Computer and Telecommunication Engineering (CTE).
more
Owing to the tireless dedication of the editor-in-chief, editorial board members, and the in-house editorial team, we are proud to announce the successful online launch of the first issue of Computer and Telecommunication Engineering.
Asia Pacific Academy of Science Pte. Ltd. (APACSCI) specializes in international journal publishing. APACSCI adopts the open access publishing model and provides an important communication bridge for academic groups whose interest fields include engineering, technology, medicine, computer, mathematics, agriculture and forestry, and environment.