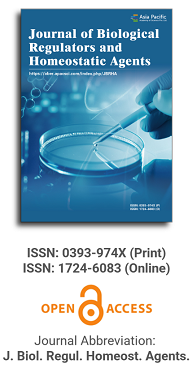
Asia Pacific Academy of Science Pte. Ltd. (APACSCI) specializes in international journal publishing. APACSCI adopts the open access publishing model and provides an important communication bridge for academic groups whose interest fields include engineering, technology, medicine, computer, mathematics, agriculture and forestry, and environment.
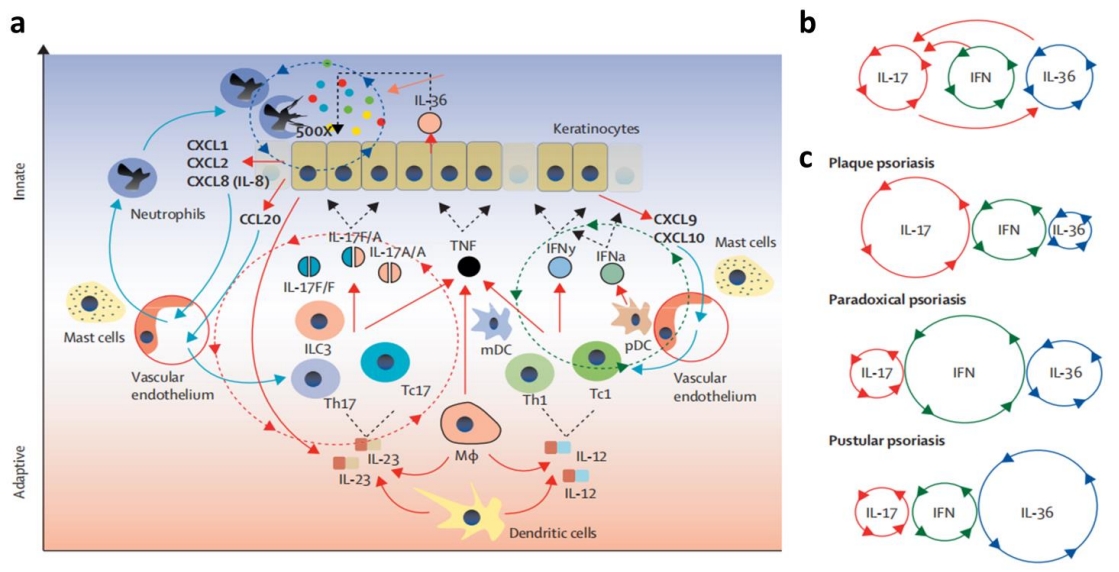
A review of glycolysis and autism spectrum disorder: The dual role of lactic acid in neurodevelopment and function
Vol 39, Issue 2, 2025
Download PDF
Abstract
Autism Spectrum Disorder (ASD) is a complex neurodevelopmental disorder characterized by impairments in social interaction, communication, and repetitive behavioral patterns. ASD is often accompanied by metabolic abnormalities, dysregulation of the immune system, and neuroinflammation. Glycolysis, a central pathway in energy metabolism, is vital for neurodevelopment and functioning. Recent studies have indicated that patients with ASD may experience disturbances in brain metabolism, particularly in the glycolytic pathway, with abnormal lactic acid production and utilization. Lactic acid serves not only as an energy source for cellular functions but also plays a significant role in cell signaling, gene expression regulation, and immune modulation. This review examines the mechanisms of glycolysis, especially the role of lactic acid in ASD; explores the relationship between lactic acid accumulation and neuroinflammation, neuroplasticity, and neurotrophic factors; and discusses the potential of lactic acid as a diagnostic and therapeutic target for ASD. Future research on modulating lactic acid metabolism may offer new strategies for the early diagnosis, precise treatment, and neural repair of ASD.
Keywords
References
- The Lancet. DSM-5: diagnosis of mental disorders. The Lancet. 2010; 376(9739): 390.
- Baio J, Wiggins L, Christensen DL, et al. Prevalence of Autism Spectrum Disorder Among Children Aged 8 Years—Autism and Developmental Disabilities Monitoring Network, 11 Sites, United States, 2014. MMWR Surveillance Summaries. 2018; 67(6): 1-23. doi: 10.15585/mmwr.ss6706a1
- Alharthi A, Alhazmi S, Alburae N, et al. The Human Gut Microbiome as a Potential Factor in Autism Spectrum Disorder. International Journal of Molecular Sciences. 2022; 23(3): 1363. doi: 10.3390/ijms23031363
- Hughes HK, Moreno RJ, Ashwood P. Innate immune dysfunction and neuroinflammation in autism spectrum disorder (ASD). Brain, Behavior, and Immunity. 2023; 108: 245-254. doi: 10.1016/j.bbi.2022.12.001
- Wang L, Wang B, Wu C, et al. Autism Spectrum Disorder: Neurodevelopmental Risk Factors, Biological Mechanism, and Precision Therapy. International Journal of Molecular Sciences. 2023; 24(3): 1819. doi: 10.3390/ijms24031819
- Ebert DH, Greenberg ME. Activity-dependent neuronal signalling and autism spectrum disorder. Nature. 2013; 493(7432): 327-337. doi: 10.1038/nature11860
- Foss-Feig JH, Adkinson BD, Ji JL, et al. Searching for Cross-Diagnostic Convergence: Neural Mechanisms Governing Excitation and Inhibition Balance in Schizophrenia and Autism Spectrum Disorders. Biological Psychiatry. 2017; 81(10): 848-861. doi: 10.1016/j.biopsych.2017.03.005
- Yoshida F, Nagatomo R, Utsunomiya S, et al. Soluble form of Lingo2, an autism spectrum disorder-associated molecule, functions as an excitatory synapse organizer in neurons. Translational Psychiatry. 2024; 14(1). doi: 10.1038/s41398-024-03167-5
- Gata-Garcia A, Diamond B. Maternal Antibody and ASD: Clinical Data and Animal Models. Frontiers in Immunology. 2019; 10. doi: 10.3389/fimmu.2019.01129
- Usui N, Kobayashi H, Shimada S. Neuroinflammation and Oxidative Stress in the Pathogenesis of Autism Spectrum Disorder. International Journal of Molecular Sciences. 2023; 24(6): 5487. doi: 10.3390/ijms24065487
- Brister D, Rose S, Delhey L, et al. Metabolomic Signatures of Autism Spectrum Disorder. Journal of Personalized Medicine. 2022; 12(10): 1727. doi: 10.3390/jpm12101727
- Gaspar JM, Carvalho HM, Camacho-Morales A. Editorial: Metabolic Disorders Associated With Autism Spectrum Disorders: Approaches for Intervention. Frontiers in Neuroscience. 2021; 15. doi: 10.3389/fnins.2021.809978
- Xie K, Sun Y, Li X, et al. Biomarkers and pathways in autism spectrum disorder: An individual meta-analysis based on proteomic and metabolomic data. European Archives of Psychiatry and Clinical Neuroscience; 2024. doi: 10.1007/s00406-024-01922-9
- Maier S, Nickel K, Lange T, et al. Increased cerebral lactate levels in adults with autism spectrum disorders compared to non-autistic controls: a magnetic resonance spectroscopy study. Molecular Autism. 2023; 14(1). doi: 10.1186/s13229-023-00577-y
- Rolfe DF, Brown GC. Cellular energy utilization and molecular origin of standard metabolic rate in mammals. Physiological Reviews. 1997; 77(3): 731-758. doi: 10.1152/physrev.1997.77.3.731
- Wei Y, Miao Q, Zhang Q, et al. Aerobic glycolysis is the predominant means of glucose metabolism in neuronal somata, which protects against oxidative damage. Nature Neuroscience. 2023; 26(12): 2081-2089. doi: 10.1038/s41593-023-01476-4
- Pellerin L, Magistretti PJ. Glutamate uptake into astrocytes stimulates aerobic glycolysis: a mechanism coupling neuronal activity to glucose utilization. Proceedings of the National Academy of Sciences. 1994; 91(22): 10625-10629. doi: 10.1073/pnas.91.22.10625
- Muraleedharan R, Gawali MV, Tiwari D, et al. AMPK-Regulated Astrocytic Lactate Shuttle Plays a Non-Cell-Autonomous Role in Neuronal Survival. Cell Reports. 2020; 32(9): 108092. doi: 10.1016/j.celrep.2020.108092
- Knaus LS, Basilico B, Malzl D, et al. Large neutral amino acid levels tune perinatal neuronal excitability and survival. Cell. 2023; 186(9): 1950-1967.e25. doi: 10.1016/j.cell.2023.02.037
- Wang Y, Li P, Xu Y, et al. Lactate metabolism and histone lactylation in the central nervous system disorders: impacts and molecular mechanisms. Journal of Neuroinflammation. 2024; 21(1). doi: 10.1186/s12974-024-03303-4
- Zhang Y, Jia P, Wang K, et al. Lactate modulates microglial inflammatory responses after oxygen-glucose deprivation through HIF-1α-mediated inhibition of NF-κB. Brain Research Bulletin. 2023; 195: 1-13. doi: 10.1016/j.brainresbull.2023.02.002
- Zhou Y, Liu X, Huang C, et al. Lactate Activates AMPK Remodeling of the Cellular Metabolic Profile and Promotes the Proliferation and Differentiation of C2C12 Myoblasts. International Journal of Molecular Sciences. 2022; 23(22): 13996. doi: 10.3390/ijms232213996
- Zhang Y, Peng Q, Zheng J, et al. The function and mechanism of lactate and lactylation in tumor metabolism and microenvironment. Genes & Diseases. 2023; 10(5): 2029-2037. doi: 10.1016/j.gendis.2022.10.006
- Wang Y, Zhao J, Zhao L. L-Lactate Administration Improved Synaptic Plasticity and Cognition in Early 3xTg-AD Mice. International Journal of Molecular Sciences. 2025; 26(4): 1486. doi: 10.3390/ijms26041486
- Wu A, Lee D, Xiong WC. Lactate Metabolism, Signaling, and Function in Brain Development, Synaptic Plasticity, Angiogenesis, and Neurodegenerative Diseases. International Journal of Molecular Sciences. 2023; 24(17): 13398. doi: 10.3390/ijms241713398
- Merkuri F, Rothstein M, Simoes-Costa M. Histone lactylation couples cellular metabolism with developmental gene regulatory networks. Nature Communications. 2024; 15(1). doi: 10.1038/s41467-023-44121-1
- Vallée A, Vallée JN. Warburg effect hypothesis in autism Spectrum disorders. Molecular Brain. 2018; 11(1). doi: 10.1186/s13041-017-0343-6
- Warburg O. On the Origin of Cancer Cells. Science. 1956; 123(3191): 309-314. doi: 10.1126/science.123.3191.309
- Han H, Zhao Y, Du J, et al. Exercise improves cognitive dysfunction and neuroinflammation in mice through Histone H3 lactylation in microglia. Immunity & Ageing. 2023; 20(1). doi: 10.1186/s12979-023-00390-4
- Khaliulin I, Hamoudi W, Amal H. The multifaceted role of mitochondria in autism spectrum disorder. Molecular Psychiatry. 2024; 30(2): 629-650. doi: 10.1038/s41380-024-02725-z
- László A, Horváth E, Eck E, et al. Serum serotonin, lactate and pyruvate levels in infantile autistic children. Clinica Chimica Acta. 1994; 229(1-2): 205-207. doi: 10.1016/0009-8981(94)90243-7
- Witters P, Debbold E, Crivelly K, et al. Autism in patients with propionic acidemia. Molecular Genetics and Metabolism. 2016; 119(4): 317-321. doi: 10.1016/j.ymgme.2016.10.009
- Correia C, Coutinho AM, Diogo L, et al. Brief Report: High Frequency of Biochemical Markers for Mitochondrial Dysfunction in Autism: No Association with the Mitochondrial Aspartate/Glutamate Carrier SLC25A12 Gene. Journal of Autism and Developmental Disorders. 2006; 36(8): 1137-1140. doi: 10.1007/s10803-006-0138-6
- Lauritzen KH, Morland C, Puchades M, et al. Lactate Receptor Sites Link Neurotransmission, Neurovascular Coupling, and Brain Energy Metabolism. Cerebral Cortex. 2013; 24(10): 2784-2795. doi: 10.1093/cercor/bht136
- Teixeira PDS, Ramos-Lobo AM, Furigo IC, et al. Brain STAT5 Modulates Long-Term Metabolic and Epigenetic Changes Induced by Pregnancy and Lactation in Female Mice. Endocrinology. 2019; 160(12): 2903-2917. doi: 10.1210/en.2019-00639
- Frye RE, Rincon N, McCarty PJ, et al. Biomarkers of mitochondrial dysfunction in autism spectrum disorder: A systematic review and meta-analysis. Neurobiology of Disease. 2024; 197: 106520. doi: 10.1016/j.nbd.2024.106520
- Goh S, Dong Z, Zhang Y, et al. Mitochondrial Dysfunction as a Neurobiological Subtype of Autism Spectrum Disorder. JAMA Psychiatry. 2014; 71(6): 665. doi: 10.1001/jamapsychiatry.2014.179
- Yehia L, Ni Y, Feng F, et al. Distinct Alterations in Tricarboxylic Acid Cycle Metabolites Associate with Cancer and Autism Phenotypes in Cowden Syndrome and Bannayan-Riley-Ruvalcaba Syndrome. The American Journal of Human Genetics. 2019; 105(4): 813-821. doi: 10.1016/j.ajhg.2019.09.004
- Manosalva C, Quiroga J, Hidalgo AI, et al. Role of Lactate in Inflammatory Processes: Friend or Foe. Frontiers in Immunology. 2022; 12. doi: 10.3389/fimmu.2021.808799
- Haas R, Smith J, Rocher-Ros V, et al. Lactate Regulates Metabolic and Pro-inflammatory Circuits in Control of T Cell Migration and Effector Functions. Marrack P, ed. PLOS Biology. 2015; 13(7): e1002202. doi: 10.1371/journal.pbio.1002202
- Zhao A, Xu W, Han R, et al. Role of histone modifications in neurogenesis and neurodegenerative disease development. Ageing Research Reviews. 2024; 98: 102324. doi: 10.1016/j.arr.2024.102324
- Brooks GA. The Science and Translation of Lactate Shuttle Theory. Cell Metabolism. 2018; 27(4): 757-785. doi: 10.1016/j.cmet.2018.03.008
- Jia L, Liao M, Mou A, et al. Rheb-regulated mitochondrial pyruvate metabolism of Schwann cells linked to axon stability. Developmental Cell. 2021; 56(21): 2980-2994.e6. doi: 10.1016/j.devcel.2021.09.013
- Peralta RM, Xie B, Lontos K, et al. Dysfunction of exhausted T cells is enforced by MCT11-mediated lactate metabolism. Nature Immunology. 2024; 25(12): 2297-2307. doi: 10.1038/s41590-024-01999-3
- Rexrode LE, Hartley J, Showmaker KC, et al. Molecular profiling of the hippocampus of children with autism spectrum disorder. Molecular Psychiatry. 2024; 29(7): 1968-1979. doi: 10.1038/s41380-024-02441-8
- Brooks GA. Lactate as a fulcrum of metabolism. Redox Biology. 2020; 35: 101454. doi: 10.1016/j.redox.2020.101454
- Huang Z, Zhang Y, Zhou R, et al. Lactate as Potential Mediators for Exercise-Induced Positive Effects on Neuroplasticity and Cerebrovascular Plasticity. Frontiers in Physiology. 2021; 12. doi: 10.3389/fphys.2021.656455
- Shang Q, Bian X, Zhu L, et al. Lactate Mediates High-Intensity Interval Training—Induced Promotion of Hippocampal Mitochondrial Function through the GPR81-ERK1/2 Pathway. Antioxidants. 2023; 12(12): 2087. doi: 10.3390/antiox12122087
- El Hayek L, Khalifeh M, Zibara V, et al. Lactate mediates the effects of exercise on learning and memory through SIRT1-dependent activation of hippocampal brain-derived neurotrophic factor (BDNF). The Journal of Neuroscience. Published online January 28, 2019: 1661-18. doi: 10.1523/jneurosci.1661-18.2019
- Feng G, Zhang L, Bao W, et al. Gentisic acid prevents colorectal cancer metastasis via blocking GPR81-mediated DEPDC5 degradation. Phytomedicine. 2024; 129: 155615. doi: 10.1016/j.phymed.2024.155615
- Tang I, Nisal A, Reed A, et al. Lipidomic profiling of mouse brain and human neuron cultures reveals a role for Mboat7 in mTOR-dependent neuronal migration. Science Translational Medicine. 2025; 17(779). doi: 10.1126/scitranslmed.adp5247
- Meeker R, Williams K. The p75 neurotrophin receptor: at the crossroad of neural repair and death. Neural Regeneration Research. 2015; 10(5): 721. doi: 10.4103/1673-5374.156967
- Allen SJ, Watson JJ, Shoemark DK, et al. GDNF, NGF and BDNF as therapeutic options for neurodegeneration. Pharmacology & Therapeutics. 2013; 138(2): 155-175. doi: 10.1016/j.pharmthera.2013.01.004
- Yang F, You H, Mizui T, et al. Inhibiting proBDNF to mature BDNF conversion leads to ASD-like phenotypes in vivo. Molecular Psychiatry. 2024; 29(11): 3462-3474. doi: 10.1038/s41380-024-02595-5
- Müller P, Duderstadt Y, Lessmann V, et al. Lactate and BDNF: Key Mediators of Exercise Induced Neuroplasticity? Journal of Clinical Medicine. 2020; 9(4): 1136. doi: 10.3390/jcm9041136
- Torres-Torrelo H, Ortega-Sáenz P, Gao L, et al. Lactate sensing mechanisms in arterial chemoreceptor cells. Nature Communications. 2021; 12(1). doi: 10.1038/s41467-021-24444-7
- Lian B, Zhang J, Yin X, et al. SIRT1 improves lactate homeostasis in the brain to alleviate parkinsonism via deacetylation and inhibition of PKM2. Cell Reports Medicine. 2024; 5(8): 101684. doi: 10.1016/j.xcrm.2024.101684
- Wang MY, Zhou Y, Li WL, et al. Friend or foe: Lactate in neurodegenerative diseases. Ageing Research Reviews. 2024; 101: 102452. doi: 10.1016/j.arr.2024.102452
- Yang H, Mo N, Tong L, et al. Microglia lactylation in relation to central nervous system diseases. Neural Regeneration Research. 2024; 20(1): 29-40. doi: 10.4103/nrr.nrr-d-23-00805
- Wang D, Jiang Y, Jiang J, et al. Gut microbial GABA imbalance emerges as a metabolic signature in mild autism spectrum disorder linked to overrepresented Escherichia. Cell Reports Medicine. 2025; 6(1): 101919. doi: 10.1016/j.xcrm.2024.101919
- Sorboni SG, Moghaddam HS, Jafarzadeh-Esfehani R, et al. A Comprehensive Review on the Role of the Gut Microbiome in Human Neurological Disorders. Clinical Microbiology Reviews. 2022; 35(1). doi: 10.1128/cmr.00338-20
- Alberghina L. The Warburg Effect Explained: Integration of Enhanced Glycolysis with Heterogeneous Mitochondria to Promote Cancer Cell Proliferation. International Journal of Molecular Sciences. 2023; 24(21): 15787. doi: 10.3390/ijms242115787
- Cai X, Ng CP, Jones O, et al. Lactate activates the mitochondrial electron transport chain independently of its metabolism. Molecular Cell. 2023; 83(21): 3904-3920.e7. doi: 10.1016/j.molcel.2023.09.034
- Dai Y, Zhang L, Yu J, et al. Improved symptoms following bumetanide treatment in children aged 3−6 years with autism spectrum disorder: a randomized, double-blind, placebo-controlled trial. Science Bulletin. 2021; 66(15): 1591-1598. doi: 10.1016/j.scib.2021.01.008
- Elnahas EM, Abuelezz SA, Mohamad MI, et al. Novel role of peroxisome proliferator activated receptor-α in valproic acid rat model of autism: Mechanistic study of risperidone and metformin monotherapy versus combination. Progress in Neuro-Psychopharmacology and Biological Psychiatry. 2022; 116: 110522. doi: 10.1016/j.pnpbp.2022.110522
- Ye N, Qin W, Tian S, et al. Small Molecules Selectively Targeting Sigma-1 Receptor for the Treatment of Neurological Diseases. Journal of Medicinal Chemistry. 2020; 63(24): 15187-15217. doi: 10.1021/acs.jmedchem.0c01192
- Goguadze N, Zhuravliova E, Morin D, et al. Sigma-1 Receptor Agonists Induce Oxidative Stress in Mitochondria and Enhance Complex I Activity in Physiological Condition but Protect Against Pathological Oxidative Stress. Neurotoxicity Research. 2017; 35(1): 1-18. doi: 10.1007/s12640-017-9838-2
- Reyes ST, Deacon RMJ, Guo SG, et al. Effects of the sigma-1 receptor agonist blarcamesine in a murine model of fragile X syndrome: neurobehavioral phenotypes and receptor occupancy. Scientific Reports. 2021; 11(1). doi: 10.1038/s41598-021-94079-7
- Zhang C, Yang L, Wang F, et al. Therapeutic Efficacy of a Synthetic Brain-Targeted H2S Donor Cross-Linked Nanomicelle in Autism Spectrum Disorder Rats through Aerobic Glycolysis. ACS Applied Materials & Interfaces. 2024; 17(1): 157-173. doi: 10.1021/acsami.4c11663
Supporting Agencies
Copyright (c) 2025 Author(s)
License URL: https://creativecommons.org/licenses/by/4.0/
This site is licensed under a Creative Commons Attribution 4.0 International License (CC BY 4.0).
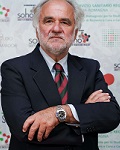
Medical Genetics, University of Torino Medical School, Italy
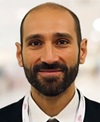
Department of Biomedical, Surgical and Dental Sciences, University of Milan, Italy