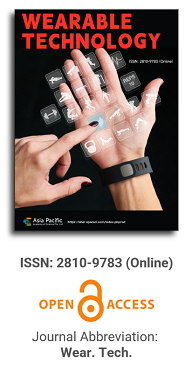
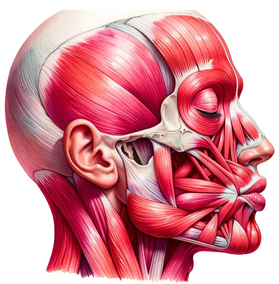
This paper delves deeply into the innovative realm of integrating human emotions with wearable technology. The primary focus is on the conceptualization and development of a kiss transfer device that harnesses the power of wearable technology to bridge the physical gap in human-human interactions. By investigating the intricate nuances of the human-human kissing process, the research seeks to replicate this intimate gesture through a technological medium. The paper not only elaborates on the anatomy, evolution, and hormonal dynamics of kissing but also underscores the transformative potential of wearable technology in capturing and transmitting these intimate moments. This exploration opens up new horizons for long-distance relationships, offering a tangible touchpoint that goes beyond traditional communication methods. Through this pioneering work, the research positions wearable technology as not just a tool for communication but as an extension of our human emotions and expressions.
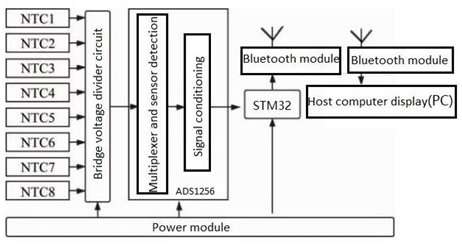
Research progress of flexible wearable stress sensor
Vol 2, Issue 2, 2021
VIEWS - 2653 (Abstract)
Download PDF
Abstract
Flexible wearable pressure sensors are widely used in health diagnosis, sports monitoring, rehabilitation medicine, entertainment, and other fields due to some factors such as the stretch ability, bendability, light weight, portability, and excellent electrical properties. In recent years, significant progress has been made in flexible pressure sensors, and a variety of flexible pressure sensors that able to measure health status have been applied to the pulse wave, movement, respiration, and electrocardiogram (ECG) detection. However, there are still many problems to be solved in the development of flexible pressure sensors. This article summarizes the development of flexible pressure sensors in recent years, from the working principle to the structural design of the flexible pressure sensors; designs to build a high-performance flexible pressure sensors; discusses the problems existing in current flexible pressure sensors and envisions the development trend of flexible pressure sensors in the future. Flexible pressure sensors with excellent flexibility, good biocompatibility, rapid response, high sensitivity, and multifunctional integration have shown a broad application prospects.
Keywords
References
1. Das R, He X. Flexible, printed and organic electronics 2020–2030: Forecasts, technologies, markets [Internet]. Cambridge: IDTechEx; [cited: 2020 Nov 9]. Available from:
2. https://www.idtechex.com/en/research-report/flexible-printed-and-organic-electronics-2020-2030-forecasts-technologies-markets/687.
3. Gao W, Ota H, Kiriya D, et al. Flexible electronics
4. toward wearable sensing. Accounts of Chemical Research 2019; 52(3): 523–533.
5. Huang Y, Fan X, Chen S, et al. Emerging technologies of flexible pressure sensors: Materials, modeling, devices, and manufacturing. Advanced Functional Materials 2019; 29(12): 1808509.
6. Trung TQ, Lee N. Flexible and stretchable physical sensor integrated platforms for wearable human-activity monitoring and personal healthcare. Advanced Materials 2016; 28(22): 4338–4372.
7. Wang L, Lou Z, Jiang K, et al. Bio-multifunctional smart wearable sensors for medical devices. Advanced Intelligent Systems 2019; 1(5): 1900040.
8. Mondal S, Zehra N, Choudhury A, et al. Wearable sensing devices for point of care diagnostics. ACS Applied Bio Materials 2021; 4(1): 47–70.
9. Cai F, Yi C, Liu S, et al. Ultrasensitive, passive and wearable sensors for monitoring human muscle motion and physiological signals. Biosensors and Bioelectronics 2016; 77: 907–913.
10. Pang Q, Lou D, Li S, et al. Smart flexible electronics-integrated wound dressing for real-time monitoring and on-demand treatment of infected wounds. Advanced Science 2020; 7(6): 1902673.
11. Lee H, Choi TK, Lee YB, et al. A graphene-based electrochemical device with thermoresponsive microneedles for diabetes monitoring and therapy. Nature Nanotechnology 2016; 11(6): 566–572.
12. Zhang H, Sun L, Liu Y. Development of flexible sensing technology in wearable medical devices. Advances in Biomedical Engineering 2020; 41(4): 201–205.
13. Yao S, Swetha P, Zhu Y. Nanomaterial-enabled wearable sensors for healthcare. Advanced Healthcare Materials 2018; 7(1): 1700889.
14. Gao W, Ota H, Kiriya D, et al. Flexible electronics toward wearable sensing. Accounts of Chemical Research 2019; 52(3): 523–533.
15. Hou X, Guo C. Principle and application of flexible pressure sensor. Acta Physica Sinica 2020; 69(17): 70–85.
16. Zhang Y, Hu Y, Zhu P, et al. Flexible and highly sensitive pressure sensor based on microdome-patterned PDMS forming with assistance of colloid self-assembly and replica technique for wearable electronics. ACS Applied Materials & Interfaces 2017; 9 (41): 35968–35976.
17. Pu J, Zha X, Tang L, et al. Human skin-inspired electronic sensor skin with electromagnetic interference shielding for the sensation and protection of wearable electronics. ACS Applied Materials & Interfaces 2018; 10(47): 40880–40889.
18. Nie B, Li X, Shao J, et al. Flexible and transparent strain sensors with embedded multiwalled carbon nanotubes meshes. ACS Applied Materials & Interfaces 2017; 9(46): 40681–40689.
19. Chen J, Zhang J, Luo Z, et al. Superelastic, sensitive, and low hysteresis flexible strain sensor based on wave-patterned liquid metal for human activity monitoring. ACS Applied Materials & Interfaces 2020; 12(19): 22200–22211.
20. Yang Y, Shi L, Cao Z, et al. Strain sensors with a high sensitivity and a wide sensing range based on a Ti3C2Tx (MXene) nanoparticle-nanosheet hybrid network. Advanced Functional Materials 2019; 29(14): 1807882.
21. Wang Y, Gong S, Gómez D, et al. Unconventional janus properties of enokitake-like gold nanowire films. ACS Nano 2018; 12(8): 8717–8722.
22. Mannsfeld SCB, Tee BC, Stoltenberg RM, et al. Highly sensitive flexible pressure sensors with mi-crostructured rubber dielectric layers. Nature Materials 2010; 9(10): 859–864.
23. He Z, Chen W, Liang B, et al. Capacitive pressure sensor with high sensitivity and fast response to dynamic interaction based on graphene and porous nylon networks. ACS Applied Materials & Interfaces 2017; 10(15): 12816–12823.
24. Kang S, Lee J, Lee S, et al. Highly sensitive pressure sensor based on bioinspired porous structure for real-time tactile sensing. Advanced Electronic Materials 2016; 2(12): 1600356.
25. Chorsi MT, Curry EJ, Chorsi HT, et al. Piezoelectric biomaterials for sensors and actuators. Advanced Materials 2019; 31(1): 1802084.
26. Wang Y, Zheng J, Ren GY, et al. A flexible piezoelectric force sensor based on PVDF fabrics. Smart Materials and Structures 2011; 20(4): 45009.
27. Park S, Lee HB, Yeon SM, et al. Flexible and stretchable piezoelectric sensor with thickness-tunable configuration of electrospun nanofiber mat and elastomeric substrates. ACS Applied Materials & Interfaces 2016; 8(37): 24773–24781.
28. HosseiniSM, Yousefi AA. Piezoelectric sensor based on electrospun PVDF-MWCNT-Cloisite 30B hybrid nanocomposites. Organic Electronics 2017; 50: 121–129.
29. Wu C, Chou M, Zeng W. Piezoelectric response of aligned electrospun polyvinylidene fluoride/carbon nanotube nanofibrous membranes. Nanomaterials 2018; 8(6): 420.
30. Wang X, Sun F, Yin G, et al. Tactile-sensing based on flexible PVDF nanofibers via electrospinning: a review. Sensors 2018; 18(2): 330.
31. Chen S, Lou Z, Chen D, et al. Highly flexible strain sensor based on ZnO nanowires and P (VDFTrFE) fibers for wearable electronic device. Science China Materials 2016; 59 (3): 173–181.
32. Liu S, Wang L, Feng X, et al. Ultrasensitive 2D ZnO piezotronic transistor array for high resolution tactile imaging. Advanced Materials 2017; 29(16): 1606346.
33. Wang J, Jiang J, Zhang C, et al. Energy-efficient, fully flexible, high-performance tactile sensor based on piezotronic effect: piezoelectric signal amplified with organic field-effect transistors. Nano Energy 2020; 76: 105050.
34. Dai X, Vo R, Hsu H, et al. Modularized field-effect transistor biosensors. Nano Letters 2019; 19(9): 6658–6664.
35. Ahmad R, Mahmoudi T, Ahn M, et al. Recent advances in nanowires-based field-effect transistors for biological sensor applications. Biosensors and Bioelectronics 2018; 100: 312–325.
36. Jiang J, Li J, Li Y, et al. Stable InSe transistors with high-field effect mobility for reliable nerve signal sensing. NPJ 2D Materials and Applications 2019; 3(1): 1–8.
37. Wu X, Han Y, Zhang X, et al. Large-area compliant, low-cost, and versatile pressure-sensing platform based on microcrack-designed carbon black@polyurethane sponge for human-machine interfacing. Advanced Functional Materials 2016; 26(34): 6246–6256.
38. Chen M, Guo H, Yang J, et al. Preparation and characteristics of metal wrinkle on flexible substrates. Science Technology and Engineering 2015; 15(12): 206–209.
39. Miyamoto A, Lee S, Cooray N F, et al. Inflammation-free, gaspermeable, lightweight, stretchable on-skin electronics with nanomeshes. Nature Nanotechnology 2017; 12(9): 907–913.
40. Han S, Kim M K, Wang B, et al. Mechanically reinforced skin-electronics with networked nanocomposite elastomer. Advanced Materials 2016; 28(46): 10257–10265.
41. Kang D, Pikhitsa PV, Choi YW, et al. Ultrasensitive mechanical crack-based sensor inspired by the spider sensory system. Nature 2014; 516(7530): 222–226.
42. Pegan JD, Zhang J, Chu M, et al. Skin-mountable stretch sensor for wearable health monitoring. Nanoscale 2016; 8(39): 17295–17303.
43. Won P, Park JJ, Lee T, et al. Stretchable and trans-parent kirigami conductor of nanowire percolation network for electronic skin applications. Nano Letters 2019; 19(9): 6087–6096.
44. Yue Y, Liu N, Liu W, et al. 3D hybrid porous Mxene-sponge network and its application in pie-zoresistive sensor. Nano Energy 2018; 50: 79–87.
45. Wang M, Zhang K, Dai X, et al. Enhanced electrical conductivity and piezoresistive sensing in multi-wall carbon nanotubes/ polydimethylsiloxane nanocomposites via the construction of a self-segregated structure. Nanoscale 2017; 9(31): 11017–11026.
46. Liu H, Li Q, Zhang S, et al. Electrically conductive polymer composites for smart flexible strain sensors: A critical review. Journal of Materials Chemistry C 2018; 6(45): 12121–12141.
47. Wu S, Peng S, Yu Y, et al. Strategies for designing stretchable strain sensors and conductors. Advanced Materials Technologies 2019; 5(2): 1900908.
48. Gong S, Yap LW, Zhu B, et al. Local crack-programmed gold nanowire electronic skin tattoos for in-plane multisensor integration. Advanced Materials 2019; 31(41): 1903789.
49. Yang T, Li X, Jiang X, et al. Structural engineering of gold thin films with channel cracks for ultrasensitive strain sensing. Materials Horizons 2016; 3(3): 248–255.
50. Liu Z, Wang X, Qi D, et al. High-adhesion stretch-able electrodes based on nanopile interlocking. Advanced Materials 2017; 29(2): 1603382.
51. Shi B, Liu Z, Zheng Q, et al. Body-integrated self-powered system for wearable and implantable applications. ACS Nano 2019; 13(5): 6017–6024.
52. Park DY, Joe DJ, Kim DH, et al. Self-powered real-time arterial pulse monitoring using ultrathin epidermal piezoelectric sensors. Advanced Materials 2017; 29(37): 1702308.
53. Li H, Han W, Jiang Y, et al. Research progress of flexible self-powered sensor. Electronic Components and Materials 2020; 39(8): 1–12.
54. Wu H, Huang Y, Xu F, et al. Energy harvesters for wearable and stretchable electronics: From flexibility to stretchability. Advanced Materials 2016; 28(45): 9881–9919.
55. Yang P, Lin Z, Pradel KC, et al. Paper-based origami triboelectric nanogenerators and self-powered pressure sensors. ACS Nano 2015; 9(1): 901–907.
56. Ma M, Zhang Z, Liao Q, et al. Self-powered artificial electronic skin for high-resolution pressure sensing. Nano Energy 2017; 32: 389–396.
57. Wang L, Lou Z, Jiang K, et al. Bio-multifunctional smart wearable sensors for medical devices. Advanced Intelligent Systems 2019; 1(5): 1900040.
58. Boutry CM, Kaizawa Y, Schroeder BC, et al. A stretchable and biodegradable strain and pressure sensor for orthopaedic application. Nature Electronics 2018; 1(5): 314–321.
59. Zhu Y, Yang B, Liu J, et al. A flexible and biocom-patible triboelectric nanogenerator with tunable in-ternal resistance for powering wearable devices. Scientific Reports 2016; 6(1): 22233.
60. Li W, Torres D, Wang T, et al. Flexible and bio-compatible polypropylene ferroelectret nanogenerator (FENG): On the path toward wearable devices powered by human motion. Nano Energy 2016; 30: 649–657.
61. Li Y, Chen W, Lu L. Wearable and biodegradable sensors for human health monitoring. ACS Applied Bio Materials 2021; 4(1): 122–139.
62. Ko G, Han SD, Kim J, et al. Biodegradable, flexible silicon nanomembrane-based NOx gas sensor system with record-high performance for transient environmental monitors and medical implants. NPG Asia Materials 2020; 12(1): 71.
63. Chung HU, Kim BH, Lee JY, et al. Binodal, wireless epidermal electronic systems with in-sensor analytics for neonatal intensive care. Science 2019; 363(6430): 780.
64. Chung HU, Rwei AY, Hourlier A, et al. Skin-interfaced biosensors for advanced wireless physiological monitoring in neonatal and pediatric intensive-care units. Nature Medicine 2020; 26(3): 418–429.
65. Wang C, Xia K, Zhang M, et al. An all-silk-derived dual-mode e-skin for simultaneous temperature-pressure detection. ACS Applied Materials & Interfaces 2017; 9(45): 39484–39492.
66. Gui Q, He Y, Gao N, et al. A skin-inspired integrated sensor for synchronous monitoring of multiparameter signals. Advanced Functional Materials 2017; 27(36): 1702050.
67. Yamamoto Y, Harada S, Yamamoto D, et al. Printed multifunctional flexible device with an integrated motion sensor for health care monitoring. Science Advances 2016; 2(11): e1601473.
68. Ray TR, Choi J, Bandodkar AJ, et al. Bio-integrated wearable systems: A comprehensive review. Chemical Reviews 2019; 119(8): 5461–5533.
Refbacks
- There are currently no refbacks.
Copyright (c) 2021 Jun Qiu, Dalong Xiang, Renqiao Wang, Haoran Wang

This work is licensed under a Creative Commons Attribution 4.0 International License.
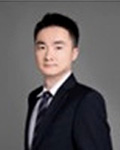
Prof. Zhen Cao
College of Information Science & Electronic Engineering, Zhejiang University
China, China
Processing Speed
-
-
-
- <5 days from submission to initial review decision;
- 62% acceptance rate
-
-
Asia Pacific Academy of Science Pte. Ltd. (APACSCI) specializes in international journal publishing. APACSCI adopts the open access publishing model and provides an important communication bridge for academic groups whose interest fields include engineering, technology, medicine, computer, mathematics, agriculture and forestry, and environment.