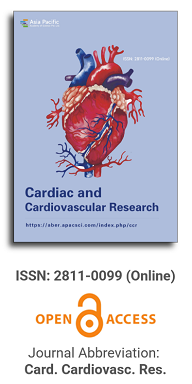
Asia Pacific Academy of Science Pte. Ltd. (APACSCI) specializes in international journal publishing. APACSCI adopts the open access publishing model and provides an important communication bridge for academic groups whose interest fields include engineering, technology, medicine, computer, mathematics, agriculture and forestry, and environment.
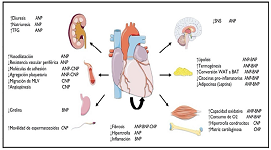
Robot-assisted coronary surgery: A narrative review on the available data
Vol 5, Issue 2, 2024
Download PDF
Abstract
The robot-based approach has been the most significant advancement in minimally invasive surgery over the past decade. Robotic coronary heart surgery represents half of the total cases of robotics-based cardiac surgery. Since 1998, it has emerged as a revolutionary approach to standard coronary surgery. However, despite its promising beginning, there has been a growing interest in the application of robotics in surgical fields other than cardiac surgery, such as urology and general surgery. In various waves of enthusiasm, single pioneers or visionary cardiac surgeons have tried to extend robotic surgery to different heart procedures, but they still struggled to practice it as a routine approach. Over the last 20 years, robotic platforms have gained importance in minimally invasive heart surgery, with proven safety and efficacy. However, despite its feasibility, safety, and efficacy, less than 0.5%–1.0% of coronary artery bypass grafting procedures are performed using a robot-assisted setup. We believe that in cardiac surgery, the time is ripe to open up new surgical strategies that are increasingly devoted to robotics, hybrid, and augmented-reality-based assistance. With this in mind, we wish to propose an excursus on the state of the art of coronary robotic surgery, its promising results, and its possible future perspectives, with a focus on the most recent achievements. This narrative minireview addresses, therefore, experiences and all aspects related to such a technique, with particular attention gained in robotic coronary revascularization, to the anaesthesiologic as well as surgical aspects, on the learning curve, patient outcome, and related costs, wishing to enlarge the portfolio of the younger generation of cardiac surgeons. In effect, according to the literature data, we are confident that robotic heart surgery is burgeoning, and the new generation of cardiac surgeons must face a gorgeous future if we invest in training and technology.
Keywords
References
- Liu J, Al’Aref SJ, Singh G, et al. An augmented reality system for image guidance of transcatheter procedures for structural heart disease. PLOS ONE. 2019; 14(7): e0219174. doi: 10.1371/journal.pone.0219174
- Linte CA, White J, Eagleson R, et al. Virtual and augmented medical imaging environments: Enabling technology for minimally invasive cardiac Interventional guidance. IEEE Reviews in Biomedical Engineering. 2010; 3: 25–47. doi: 10.1109/rbme.2010.2082522
- Pugin F, Bucher P, Morel P. History of robotic surgery: From AESOP® and ZEUS® to da Vinci®. Journal of Visceral Surgery. 2011; 148(5): e3–e8. doi: 10.1016/j.jviscsurg.2011.04.007
- Abbou CC, Hoznek A, Salomon L, et al. Remote laparoscopic radical prostatectomy carried out with a robot. Report of a case (French). Prog Urol. 2000; 10(4): 520–3.
- Yanagawa F, Perez M, Bell T, et al. Critical outcomes in nonrobotic vs robotic-assisted cardiac surgery. JAMA Surgery. 2015; 150(8): 771–777. doi: 10.1001/jamasurg.2015.1098
- Shahin GMM, Bruinsma GJBB, Stamenkovic S, Cuesta MA. Training in robotic thoracic surgery—the European way. Annals of Cardiothoracic Surgery. 2019; 8(2): 202–209. doi: 10.21037/acs.2018.11.06
- Cerfolio RJ, Cichos KH, Wei B, et al. Robotic lobectomy can be taught while maintaining quality patient outcomes. The Journal of Thoracic and Cardiovascular Surgery. 2016; 152(4): 991–997. doi: 10.1016/j.jtcvs.2016.04.085
- Endo Y, Nakamura Y, Kuroda M, et al. The utility of a 3D endoscope and robot-assisted system for MIDCAB. Annals of Thoracic and Cardiovascular Surgery. 2019; 25(4): 200–204. doi: 10.5761/atcs.oa.18-00254
- Lewis CTP, Stephens RL, Tyndal CM, Cline JL. Concomitant robotic mitral and tricuspid valve repair: Technique and early experience. The Annals of Thoracic Surgery. 2014; 97(3): 782–787. doi: 10.1016/j.athoracsur.2013.09.049
- Ju MH, Huh JH, Lee CH, et al. Robotic-assisted surgical ablation of atrial fibrillation combined with mitral valve surgery. The Annals of Thoracic Surgery. 2019; 107(3): 762–768. doi: 10.1016/j.athoracsur.2018.08.059
- Rillig A, Schmidt B, Biase LD, et al. Manual versus robotic catheter ablation for the treatment of atrial fibrillation: The man and machine trial. JACC: Clinical Electrophysiology. 2017; 3(8): 875–883. doi: 10.1016/j.jacep.2017.01.024
- Ward AF, Applebaum RM, Toyoda N, et al. Totally endoscopic robotic left atrial appendage closure demonstrates high success rate. Innovations: Technology and Techniques in Cardiothoracic and Vascular Surgery. 2017; 12(1): 46–49. doi: 10.1097/imi.0000000000000330
- Lewis CTP, Stephens RL, Horst VD, et al. Application of an epicardial left atrial appendage occlusion device by a robotic-assisted, right-chest approach. The Annals of Thoracic Surgery. 2016; 101(5): e177–e178. doi: 10.1016/j.athoracsur.2015.11.028
- Xiao C, Gao C, Yang M, et al. Totally robotic atrial septal defect closure: 7-year single-institution experience and follow-up. Interactive CardioVascular and Thoracic Surgery. 2014; 19(6): 933–937. doi: 10.1093/icvts/ivu263
- Onan B, Aydin U, Kadirogullari E, et al. Robotic repair of partial anomalous pulmonary venous connection: the initial experience and technical details. Journal of Robotic Surgery. 2019; 14(1): 101–107. doi: 10.1007/s11701-019-00943-0
- Onan B, Aydin U, Turkvatan A, et al. Robot-assisted repair of right partial anomalous pulmonary venous return. Journal of Cardiac Surgery. 2016; 31(6): 394–397. doi: 10.1111/jocs.12753
- Lewis CTP, Bethencourt DM, Stephens RL, et al. Robotic repair of sinus venosus atrial septal defect with partial anomalous pulmonary venous return and persistent left superior vena cava. Innovations: Technology and Techniques in Cardiothoracic and Vascular Surgery. 2014; 9(5): 388–390. doi: 10.1097/imi.0000000000000093
- Onan B, Aydin U, Basgoze S, et al. Totally endoscopic robotic repair of coronary sinus atrial septal defect. Interactive CardioVascular and Thoracic Surgery. 2016; 23(4): 662–664. doi: 10.1093/icvts/ivw200
- Bakir I, Onan B, Kadirogullari E. Robotically assisted repair of partial atrioventricular canal defect. Artificial Organs. 2016; 40(9): 917–918. doi: 10.1111/aor.12800
- Gao C, Yang M, Wang G, et al. Totally endoscopic robotic ventricular septal defect repair. Innovations: Technology and Techniques in Cardiothoracic and Vascular Surgery. 2010; 5(4): 278–280. doi: 10.1097/imi.0b013e3181ee94cb
- Gao C, Yang M, Wang G, et al. Totally robotic resection of myxoma and atrial septal defect repair. Interactive Cardio Vascular and Thoracic Surgery. 2008; 7(6): 947–950. doi: 10.1510/icvts.2008.185991
- Murphy ET. Robotic excision of aortic valve papillary fibroelastoma and concomitant maze procedure. Global Cardiology Science and Practice. 2013; 2012(2): 93–100. doi: 10.5339/gcsp.2012.27
- Woo YJ, Grand TJ, Weiss SJ. Robotic resection of an aortic valve papillary fibroelastoma. The Annals of Thoracic Surgery. 2005; 80(3): 1100–1102. doi: 10.1016/j.athoracsur.2004.02.108
- Folliguet TA, Vanhuyse F, Magnano D, et al. Robotic aortic valve replacement: Case report. The Heart Surgery Forum. 2004; 7(6): E551–E553. doi: 10.1532/hsf98.20041025
- Folliguet TA, Vanhuyse F, Konstantinos Z, et al. Early experience with robotic aortic valve replacement. European Journal of Cardio-Thoracic Surgery. 2005; 28(1): 172–173. doi: 10.1016/j.ejcts.2005.03.021
- Balkhy HH, Lewis CTP, Kitahara H. Robot‐assisted aortic valve surgery: State of the art and challenges for the future. The International Journal of Medical Robotics and Computer Assisted Surgery. 2018; 14(4): e1913. doi: 10.1002/rcs.1913
- Malhotra SP, Le D, Thelitz S, et al. Robotic-assisted endoscopic thoracic aortic anastomosis in juvenile lambs. The Heart Surgery Forum. 2002; 6(1): 38–42. doi: 10.1532/hsf.879
- Srivastava SP, Patel KN, Skantharaja R, et al. Off-pump complete revascularization through a left lateral thoracotomy (ThoraCAB): The first 200 cases. The Annals of Thoracic Surgery. 2003; 76(1): 46–49.
- Neumann FJ, Sousa-Uva M, Ahlsson A, et al. 2018 ESC/EACTS Guidelines on myocardial revascularization. EuroIntervention. 2019; 14(14): 1435–1534. doi: 10.4244/EIJY19M01_01
- Patel MR, Calhoon JH, Dehmer GJ, et al. Correction to: ACC/AATS/AHA/ASE/ASNC/SCAI/SCCT/STS 2017 appropriate use criteria for coronary revascularization in patients with stable ischemic heart disease. Journal of Nuclear Cardiology. 2018; 25(6): 2191–2192. doi: 10.1007/s12350-018-1292-x
- Patel MR, Calhoon JH, Dehmer GJ, et al. ACC/AATS/AHA/ASE/ASNC/SCAI/SCCT/STS 2016 appropriate use criteria for coronary revascularization in patients with acute coronary syndromes. Journal of the American College of Cardiology. 2017; 69(5): 570–591. doi: 10.1016/j.jacc.2016.10.034
- Tajstra M, Hrapkowicz T, Hawranek M, et al. Hybrid coronary revascularization in selected patients with multivessel disease. JACC: Cardiovascular Interventions. 2018; 11(9): 847–852. doi: 10.1016/j.jcin.2018.01.271
- Guan Z, Zhang Z, Gu K, et al. Minimally invasive CABG or hybrid coronary revascularization for multivessel coronary diseases: Which is best? A Systematic Review and Metaanalysis. The Heart Surgery Forum. 2019; 22(6): E493–E502. doi: 10.1532/hsf.2499
- Gorki H, Patel NC, Balacumaraswami L, et al. Long-term survival after minimal invasive direct coronary artery bypass (MIDCAB) surgery in patients with low ejection fraction. Innovations: Technology and Techniques in Cardiothoracic and Vascular Surgery. 2010; 5(6): 400–406. doi: 10.1177/155698451000500604
- Dhawan R, Roberts JD, Wroblewski K, et al. Multivessel beating heart robotic myocardial revascularization increases morbidity and mortality. The Journal of Thoracic and Cardiovascular Surgery. 2012; 143(5): 1056–1061. doi: 10.1016/j.jtcvs.2011.06.023
- Wang S, Zhou J, Cai JF. Traditional coronary artery bypass graft versus totally endoscopic coronary artery bypass graft or robot-assisted coronary artery bypass graft--meta-analysis of 16 studies. Eur Rev Med Pharmacol Sci. 2014;18(6): 790–7.
- Srivastava S, Barrera R, Quismundo S. One One hundred sixty-four consecutive beating heart totally endoscopic coronary artery bypass cases without intraoperative conversion. The Annals of Thoracic Surgery. 2012; 94(5): 1463–1468. doi: 10.1016/j.athoracsur.2012.05.028
- Bonatti JO, Zimrin D, Lehr EJ, et al. Hybrid coronary revascularization using robotic totally endoscopic surgery: Perioperative outcomes and 5-year results. The Annals of Thoracic Surgery. 2012; 94(6): 1920–1926. doi: 10.1016/j.athoracsur.2012.05.041
- Bonaros N, Schachner T, Lehr E, et al. Five hundred cases of robotic totally endoscopic coronary artery bypass grafting: Predictors of success and safety. The Annals of Thoracic Surgery. 2013; 95(3): 803–812. doi: 10.1016/j.athoracsur.2012.09.071
- Bonaros N, Schachner T, Kofler M, et al. Advanced hybrid closed chest revascularization: an innovative strategy for the treatment of multivessel coronary artery disease. European Journal of Cardio-Thoracic Surgery. 2014; 46(6): e94–e102. doi: 10.1093/ejcts/ezu357
- Cavallaro P, Rhee AJ, Chiang Y, et al. In-hospital mortality and morbidity after robotic coronary artery surgery. Journal of Cardiothoracic and Vascular Anesthesia. 2015; 29(1): 27–31. doi: 10.1053/j.jvca.2014.03.009
- Zaouter C, Imbault J, Labrousse L, et al. Association of robotic totally endoscopic coronary artery bypass graft surgery associated with a preliminary cardiac enhanced recovery after surgery program: A retrospective analysis. Journal of Cardiothoracic and Vascular Anesthesia. 2015; 29(6): 1489–1497. doi: 10.1053/j.jvca.2015.03.003
- Kofler M, Schachner T, Sebastian JR, et al. Comparative analysis of perioperative and mid-term results of TECAB and MIDCAB for revascularization of anterior wall. Innovations: Technology and Techniques in Cardiothoracic and Vascular Surgery. 2017; 12(3): 207–213. doi: 10.1097/imi.0000000000000378
- Alaj E, Seidiramool V, Ciobanu V, et al. Short-term clinical results of minimally invasive direct coronary artery bypass (MIDCAB) procedure. Journal of Clinical Medicine. 2024; 13(11): 3124. doi: 10.3390/jcm13113124
- Algoet M, Verbelen T, Jacobs S, et al. Robot-assisted MIDCAB using bilateral internal thoracic artery: A propensity score–matched study with OPCAB patients. Innovations: Technology and Techniques in Cardiothoracic and Vascular Surgery. 2024; 19(2): 184–191. doi: 10.1177/15569845241245422
- Weymann A, Amanov L, Beltsios E, et al. Minimally invasive direct coronary artery bypass grafting: Sixteen years of single-center experience. Journal of Clinical Medicine. 2024; 13(11): 3338. doi: 10.3390/jcm13113338
- Vassiliades TA, Nielsen JL, Lonquist JL. Effects of obesity on outcomes in endoscopically assisted coronary artery bypass operations. The Heart Surgery Forum. 2003; 6(2): 99–101. doi: 10.1532/hsf.569
- Hemli JM, Darla LS, Panetta CR, et al. Does body mass index affect outcomes in robotic-assisted coronary artery bypass procedures? Innovations: Technology and Techniques in Cardiothoracic and Vascular Surgery. 2012; 7(5): 350–353. doi: 10.1097/imi.0b013e31827e1ea9
- Balacumaraswami L, Patel NC, Gorki H, et al. Minimally invasive direct coronary artery bypass as a primary strategy for reoperative myocardial revascularization. Innovations: Technology and Techniques in Cardiothoracic and Vascular Surgery. 2010; 5(1): 22–27. doi: 10.1097/imi.0b013e3181cef8a6
- Cheng Y, Liu X, Zhao Y, et al. Risk factors for postoperative events in patients on antiplatelet therapy undergoing off-pump coronary artery bypass grafting surgery. Angiology. 2020; 71(8): 704–712. doi: 10.1177/0003319720919319
- Kon ZN, Brown EN, Tran R, et al. Simultaneous hybrid coronary revascularization reduces postoperative morbidity compared with results from conventional off-pump coronary artery bypass. The Journal of Thoracic and Cardiovascular Surgery. 2008; 135(2): 367–375. doi: 10.1016/j.jtcvs.2007.09.025
- Patel NC, Hemli JM, Kim MC, et al. Short- and intermediate-term outcomes of hybrid coronary revascularization for double-vessel disease. The Journal of Thoracic and Cardiovascular Surgery. 2018; 156(5): 1799–1807.e3. doi: 10.1016/j.jtcvs.2018.04.078
- Sardar P, Kundu A, Bischoff M, et al. Hybrid coronary revascularization versus coronary artery bypass grafting in patients with multivessel coronary artery disease: A meta‐analysis. Catheterization and Cardiovascular Interventions. 2018; 91(2): 203–212. doi: 10.1002/ccd.27098
- Hemli JM, Patel NC. Robotic cardiac surgery. Surgical Clinics of North America. 2020; 100(2): 219–236. doi: 10.1016/j.suc.2019.12.005
- Loulmet D, Carpentier A, d’Attellis N, et al. Endoscopic coronary artery bypass grafting with the aid of robotic assisted instruments. The Journal of Thoracic and Cardiovascular Surgery. 1999; 118(1): 4–10. doi: 10.1016/S0022-5223(99)70133-9
- Mohr FW, Falk V, Diegeler A, et al. Computer-enhanced coronary artery bypass surgery. The Journal of Thoracic and Cardiovascular Surgery. 1999; 117(6): 1212–1214. doi: 10.1016/S0022-5223(99)70261-8
- Göbölös L, Ramahi J, Obeso A, et al. Robotic totally endoscopic coronary artery bypass grafting: Systematic review of clinical outcomes from the past two decades. Innovations: Technology and Techniques in Cardiothoracic and Vascular Surgery. 2019; 14(1): 5–16. doi: 10.1177/1556984519827703
- Caimmi PPR, Fossaceca R, Lanfranchi M, et al. Cardiac Cardiac angio-CT scan for planning MIDCAB. The Heart Surgery Forum. 2004; 7(2): E113–6. doi: 10.1532/hsf98.200328101
- Moodley S, Schoenhagen P, Gillinov AM, et al. Preoperative multidetector computed tomograpy angiography for planning of minimally invasive robotic mitral valve surgery: Impact on decision making. The Journal of Thoracic and Cardiovascular Surgery. 2013; 146(2): 262–268. doi: 10.1016/j.jtcvs.2012.06.052
- Morris MF, Suri RM, Akhtar NJ, et al. Computed tomography as an alternative to catheter angiography prior to robotic mitral valve repair. The Annals of Thoracic Surgery. 2013; 95(4): 1354–1359. doi: 10.1016/j.athoracsur.2012.12.010
- Leonard JR, Henry M, Rahouma M, et al. Systematic preoperative CT scan is associated with reduced risk of stroke in minimally invasive mitral valve surgery: A meta-analysis. International Journal of Cardiology. 2019; 278: 300–306. doi: 10.1016/j.ijcard.2018.12.025
- Fitzgerald MM, Bhatt HV, Schuessler ME, et al. Robotic Cardiac Surgery Part Ⅰ: Anesthetic Considerations in Totally Endoscopic Robotic Cardiac Surgery (TERCS). Journal of Cardiothoracic and Vascular Anesthesia. 2020; 34(1): 267–277. doi: 10.1053/j.jvca.2019.02.039
- Bhatt HV, Schuessler ME, Torregrossa G, et al. Robotic cardiac surgery Part Ⅱ: Anesthetic considerations for robotic coronary artery bypass grafting. Journal of Cardiothoracic and Vascular Anesthesia. 2020; 34(9): 2484–2491. doi: 10.1053/j.jvca.2019.11.005
- Oehlinger A, Bonaros N, Schachner T, et al. Robotic endoscopic left internal mammary artery harvesting: What have we learned after 100 cases? The Annals of Thoracic Surgery. 2007; 83(3): 1030–1034. doi: 10.1016/j.athoracsur.2006.10.055
- Bonatti J, Schachner T, Bernecker O, et al. Robotic totally endoscopic coronary artery bypass: program development and learning curve issues. The Journal of Thoracic and Cardiovascular Surgery. 2004; 127(2): 504–510. doi: 10.1016/j.jtcvs.2003.09.005
- Kappert U, Cichon R, Schneider J, et al. Robotic coronary artery surgery–the evolution of a new minimally-invasive approach in coronary artery surgery. The Thoracic and Cardiovascular Surgeon. 2000; 48(4): 193–197. doi: 10.1055/s-2000-6904
- Hemli JM, Henn LW, Panetta CR, et al. Defining the learning curve for robotic-assisted endoscopic harvesting of the left internal mammary artery. Innovations: Technology and Techniques in Cardiothoracic and Vascular Surgery. 2013; 8(5): 353–358. doi: 10.1097/imi.0000000000000017
- Yanagawa F, Perez M, Bell T, et al. Critical outcomes in nonrobotic vs robotic assisted cardiac surgery. JAMA Surgery. 2015; 150(8): 771. doi: 10.1001/jamasurg.2015.1098
- Barbash GI, Glied SA. New technology and health care costs–the case of robot-assisted surgery. New England Journal of Medicine. 2010; 363(8): 701–704. doi: 10.1056/nejmp1006602
- Whellan DJ, McCarey MM, Taylor BS, et al. Trends in robotic-assisted coronary artery bypass grafts: A study of the society of thoracic surgeons adult cardiac surgery database, 2006 to 2012. The Annals of Thoracic Surgery. 2016; 102(1): 140–146. doi: 10.1016/j.athoracsur.2015.12.059
- Balkhy HH, Amabile A, Torregrossa G. A shifting paradigm in robotic heart surgery: From single-procedure approach to establishing a robotic heart center of excellence. Innovations: Technology and Techniques in Cardiothoracic and Vascular Surgery. 2020; 15(3): 187–194. doi: 10.1177/1556984520922933
- Brown LM, Kratz A, Verba S, et al. Pain and opioid use after thoracic surgery: Where we are and where we need to go. The Annals of Thoracic Surgery. 2020; 109(6): 1638–1645. doi: 10.1016/j.athoracsur.2020.01.056
- Pirelli L, Patel NC, Scheinerman JS, et al. Hybrid minimally invasive approach for combined obstructive coronary artery disease and severe aortic stenosis. Innovations: Technology and Techniques in Cardiothoracic and Vascular Surgery. 2020; 15(2): 131–137. doi: 10.1177/1556984519896581
- Kobayashi J, Shimahara Y, Fujita T, et al. Early results of simultaneous transaortic transcatheter aortic valve implantation and total arterial off-pump coronary artery revascularization in high-risk patients. Circulation Journal. 2016; 80(9): 1946–1950. doi: 10.1253/circj.cj-16-0329
- Giustino G, Serruys PW, Sabik JF, et al. Mortality after repeat revascularization following PCI or CABG for left main disease. JACC: Cardiovascular interventions. 2020; 13(3): 375–387. doi: 10.1016/j.jcin.2019.09.019
- Baquero GA, Azarrafiy R, de Marchena EJ, et al. Hybrid off‐pump coronary artery bypass grafting surgery and transaortic transcatheter aortic valve replacement: Literature review of a feasible bailout for patients with complex coronary anatomy and poor femoral access. Journal of Cardiac Surgery. 2019; 34(7): 591–597. doi: 10.1111/jocs.14082
- Ahad S, Wachter K, Rustenbach C, et al. Concomitant therapy: Off-pump coronary revascularization and transcatheter aortic valve implantation. Interactive Cardio Vascular and Thoracic Surgery. 2017; 25(1): 12–17. doi: 10.1093/icvts/ivx029
- Falk V, Baumgartner H, Bax JJ, et al. 2017 ESC/EACTS Guidelines for the management of valvular heart disease. Eur J Cardiothorac Surg. 2017; 52(4): 616–664.
- Baumgartner H, Falk V, Bax JJ, et al. 2017 ESC/EACTS Guidelines for the management of valvular heart disease. European Heart Journal. 2017; 38(36): 2739–2791.
- Sutter FP, Wertan MC, Spragan D, et al. Robotic-assisted coronary artery bypass grafting: how I teach it. Annals of Cardiothoracic Surgery. 2024; 13(4): 346–353. doi: 10.21037/acs-2024-rcabg-0033
- Mori M, Geirsson A. The way forward in research on robotic cardiac surgery: the need for transatlantic robotic cardiac surgery registry. Annals of Cardiothoracic Surgery. 2024; 13(4): 376–378. doi: 10.21037/acs-2023-rcabg-0183
- Gianoli M, de Jong AR, van der Harst P, et al. Cost analysis of robot-assisted versus on-pump and off-pump coronary artery bypass grafting: A single-center surgical and 30-day outcomes comparison. Innovations: Technology and Techniques in Cardiothoracic and Vascular Surgery. 2024; 19(4): 416–424. doi: 10.1177/15569845241269312
- Dokollari A, Sicouri S, Prendergrast G, et al. Robotic-assisted versus traditional full-sternotomy coronary artery bypass grafting procedures: A propensity-matched analysis of hospital costs. The American Journal of Cardiology. 2024; 213: 12–19. doi: 10.1016/j.amjcard.2023.10.083
Refbacks
- There are currently no refbacks.
Copyright (c) 2024 Francesca D’Auria, Danilo Flavio Santo
License URL: https://creativecommons.org/licenses/by/4.0/
This site is licensed under a Creative Commons Attribution 4.0 International License (CC BY 4.0).
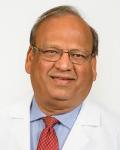
Prof. Prakash Deedwania
University of California,
San Francisco, United States

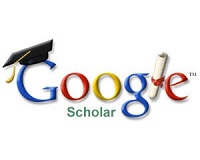
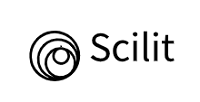