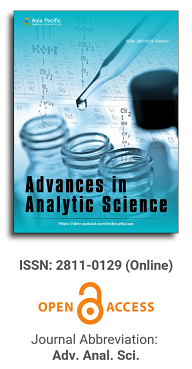
Asia Pacific Academy of Science Pte. Ltd. (APACSCI) specializes in international journal publishing. APACSCI adopts the open access publishing model and provides an important communication bridge for academic groups whose interest fields include engineering, technology, medicine, computer, mathematics, agriculture and forestry, and environment.
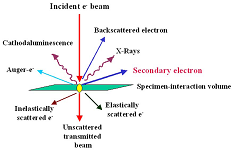
Study of the possibilities of determining mobile sulfur by physical-chemical and chemical methods in various substrates in the presence of orthanilic K
Vol 5, Issue 2, 2024
Download PDF
Abstract
The article presents the results of testing the developed method for determining mobile sulfur in wood ash and various soil substrates. Determination of sulfur in sulfate form in presence of orthanilic K is possible due to the indicator’s ability to form coloured complexes with Ba2+ ion. We examined the ranges of volumetric and photometric determination. The accuracy of the analysis was determined by comparison with a certified method. We present optimal conditions for the successful determination of the mobile sulfur with the titration and with spectrophotometric method. The results of experiments confirming the capabilities of the method under study are presented.
Keywords
References
- Luo L, Hui X, He G, et al. Benefits and Limitations to plastic mulching and nitrogen fertilization on grain yield and sulfur nutrition: Multi-site field trials in the semiarid area of China. Frontiers in Plant Science. 2022; 13: 799093. doi:10.3389/fpls.2022.799093
- Zenda T, Liu S, Dong A, et al. Revisiting sulphur – the once neglected nutrient: It’s roles in plant growth, metabolism, stress tolerance and crop production. Agriculture. 2021; 11(7): 626. doi: 10.3390/agriculture11070626
- Komarnisky LA, Christopherson RJ, Basu TK. Sulfur: Its clinical and toxicologic aspects. Nutrition. 2003; 1(19): 54-61. doi: 10.1016/S0899-9007(02)00833-X
- Hinckley ELS, Matson PA. Transformations, transport, and potential unintended consequences of high sulfur inputs to Napa Valley vineyards. Proceedings of the National Academy of Sciences. 2011; 34(108): 14005-14010. doi: 10.1073/pnas.1110741108
- Aristarkhov A. Sulfur in Russian agroecosystems: monitoring the content in soils and the effectiveness of its use. International Agricultural Journal. 2016; 5: 39-47.
- Glebova IV, Tutova OA. Agrochemical assessment of the sulfur content in the soils of the Kursk region and the feasibility of application. Agrochemistry. 2020; 4: 11-19.
- Lukin SV, Zhuikov DV, Monitoring of sulfur content in soils, plants and organic fertilizers. Agriculture. 2019; 2: 10-12. doi: 10.24411/0044-3913-2019-10202
- Scherer HW. Sulfur in soils. Journal of Plant Nutrition and Soil Science. 2009; 172: 326-335. doi: 10.1002/jpln.200900037
- Sharma RK, Cox MS, Oglesby C, et al. Revisiting the role of sulfur in crop production: A narrative review. Journal of Agriculture and Food Research. 2024; 15: 101013. doi: 10.1016/j.jafr.2024.101013
- GOST Russian Federal Standard 26490. Soils. Determination of mobile sulfur by CINAO method. USSR State Committee for Standards; 1975.
- Senyavin BF, Myasoedov MM. Determination of standardized components in external and waste water. Moscow; 1987. 197p.
- State Committee of The Russian Federation for Environmental Protection. Federal environmental regulation document 14.1:2.107-97. Method for measuring mass concentrations of sulfates in samples of natural and treated wastewater by titration with barium salt in the presence of orthanilic K. Quantitative chemical analysis of water. Moscow. The Constitution of the Russian Federation; 2005. 15p.
- Yurovskaya MA. Methods of synthesis and chemical properties of aromatic heterocyclic compounds. Moscow University Press; 2005. 34p.
- Benkhaya S, M’rabet S, El Harfi A. Classifications, properties, recent synthesis and applications of azo dyes. Heliyon. 2020; 6(1): 26. doi: 10.1016/j.heliyon.2020.e03271
- Ganjoo R, Verma C, Kumar A, et al. Colloidal and interface aqueous chemistry of dyes: Past, present and future scenarios in corrosion mitigation. Advances in Colloid and Interface Science. 2023; 311: 102832. doi: 10.1016/j.cis.2022.102832
- Arabov MSh. Metal indicators for barium ion when determining sulfate ion in water. Ecology of Industrial Production. 2003; 4: 42-22.
- Dedkov YM, Korsakova NV, Sychkova VA. New Metallochromic Indicator for Barium: Determination of Sulfate in Water and Soil Extracts. Journal of Analytical Chemistry. 2006; 61(12): 1154-1162. doi:10.1134/S1061934806120057
- Reshetnyak EA, Asmolov VE, Nemets NN, et al. Optical sensor based on hardened gelatin gel for the photometric determination of sulfates in aquatic solutions. Vestnik of Kharkov National University. Chemistry. 2010; 18(41): 74–81.
- Loginov YM, Kuznetsov VV. New method for the quantitative determination of sulfate ions in aqueous media and water extracts from soils and analytical equipment for its implementation. Agrochemistry. 2019; 10: 85-96. doi: 10.1134/S0002188119100107
- Shvoeva OP, Dedkova VP, Savvin SB. Potentialities of fibrous ion-exchange materials in the determination of sulfate ions by color reactions of barium with organic reagents on a solid phase. Journal of Analytical Chemistry. 2008; 7(63): 622-625. doi: 10.1134/S1061934808070034
- Dedkova VP, Shvoeva OP, Savvin SB. Complexation of alkaline-earth metals with bisazosubstituted chromotropic acid derivatives on fibrous ion exchanges. Journal of Analytical Chemistry. 2011; 66(1): 16-21. doi: 10.1134/S1061934811010035
- Arabov MSh. Development of a methodology for determining inorganic formers in environmental objects [PhD thesis]. Mendeleev Russian University of Chemical Technology; 2004.
- Sorokina KV. Photometric determination of mobile sulfur in the presence of orthanilic K. In: Proceedings of the Modern Problems of Natural Sciences and Pharmacy; 16–19 May 2023; Yoshkar-Ola, Russia. pp. 533-536.
Refbacks
- There are currently no refbacks.
Copyright (c) 2024 Svetlana Lokhanina, Katerina Sorokina
License URL: https://creativecommons.org/licenses/by/4.0/
This site is licensed under a Creative Commons Attribution 4.0 International License (CC BY 4.0).
1.jpg)
Prof. Sivanesan Subramanian
Anna University, India