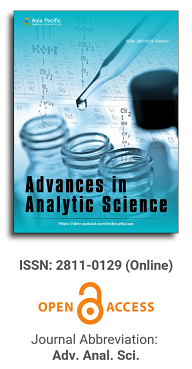
Asia Pacific Academy of Science Pte. Ltd. (APACSCI) specializes in international journal publishing. APACSCI adopts the open access publishing model and provides an important communication bridge for academic groups whose interest fields include engineering, technology, medicine, computer, mathematics, agriculture and forestry, and environment.
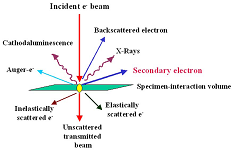
TLS noise reduction in microwave kinetic inductance detectors using a parallel plate capacitor with a three-layer dielectric
Vol 5, Issue 2, 2024
VIEWS - 1897 (Abstract)
Download PDF
Abstract
Over the past two decades, microwave kinetic inductance detectors (MKIDs) have gained exceptional importance in millimeter and sub-millimeter astronomy. MKIDs consist of thin strip resonators capable of detecting changes in the surface impedance of superconductor strips, which result from variations in resonance circuit properties. The principal noise in MKIDs comprises excess frequency noise and two-level system noise. In this paper, we propose a technique to mitigate the effect of two-level system (TLS) noise in MKIDs using a parallel plate capacitor with three layers of high ε dielectrics. To achieve this, we employ three layers of Al2O3, HfO2, and TiO2 with equal thickness between the capacitor plates. The experimental results demonstrate a nearly 30% reduction in TLS power spectral density.
Keywords
Full Text
References
1. Mazin BA, Bailey J, Bartlett J, et al. Optical and Near-IR Microwave Kinetic Inductance Detectors (MKIDs) in the 2020s. Bulletin of the American Astronomical Society. 2019; 51(7).
2. Day PK, LeDuc HG, Mazin BA, et al. A broadband superconducting detector suitable for use in large arrays. Nature. 2003; 425(6960): 817-821. doi: 10.1038/nature02037.
3. D’Addabbo A, Bellini F, Cardani L, et al. High-sensitivity Kinetic Inductance Detectors for CALDER. arXiv. 2017; arXiv:1705.04483.
4. Miller JM, Zobrist N, Ulbricht G, et al. Improving the energy resolution of photon counting microwave kinetic inductance detectors using principal component analysis. Journal of Astronomical Telescopes, Instruments, and Systems. 2021; 7(04). doi: 10.1117/1.jatis.7.4.048003.
5. Vissers MR, Austermann JE, Malnou M, et al. Ultrastable millimeter-wave kinetic inductance detectors. Applied Physics Letters. 2020; 116(3). doi: 10.1063/1.5138122
6. Mazin BA. Microwave Kinetic Inductance Detectors [PhD thesis]. California Institute of Technology; 2004.
7. Stevenson TR, Adams JS, Wen-Ting Hsieh, et al. Superconducting Films for Absorber-Coupled MKID Detectors for Sub-Millimeter and Far-Infrared Astronomy. IEEE Transactions on Applied Superconductivity. 2009; 19(3): 561-564. doi: 10.1109/tasc.2009.2019661
8. Shafiee M, Fedorov D, Grossan B, et al. A readout system for microwave kinetic inductance detectors using software defined radios. Journal of Instrumentation. 2021; 16(07): P07015. doi: 10.1088/1748-0221/16/07/p07015
9. Ishino H, Kibayashi A, Hattori K, et al. Development of Microwave Kinetic Inductance Detectors for a Detection of Phonons. Journal of Low Temperature Physics. 2013; 176(3-4): 161-167. doi: 10.1007/s10909-013-1025-0
10. Guruswamy T. Nonequilibrium behaviour and quasiparticle heating in thin film superconducting microwave resonators [PhD thesis]. University of Cambridge; 2018.
11. Ulbricht G, De Lucia M, Baldwin E. Applications for Microwave Kinetic Induction Detectors in Advanced Instrumentation. Applied Sciences. 2021; 11(6): 2671. doi: 10.3390/app11062671
12. Calvo M, Giordano C, Battiston R, et al. Development of Kinetic Inductance Detectors for Cosmic Microwave Background experiments. Experimental Astronomy. 2010; 28(2-3): 185-194. doi: 10.1007/s10686-010-9197-y
13. Ishitsuka H, Ikeno M, Oguri S, et al. Front–End Electronics for the Array Readout of a Microwave Kinetic Inductance Detector Towards Observation of Cosmic Microwave Background Polarization. Journal of Low Temperature Physics. 2016; 184(1-2): 424-430. doi: 10.1007/s10909-015-1467-7
14. Dober B, Austermann JA, Beall JA, et al. Optical Demonstration of THz, Dual-Polarization Sensitive Microwave Kinetic Inductance Detectors. Journal of Low Temperature Physics. 2015; 184(1-2): 173-179. doi: 10.1007/s10909-015-1434-3
15. Hu J, Salatino M, Traini A, et al. Proximity-Coupled Al/Au Bilayer Kinetic Inductance Detectors. Journal of Low Temperature Physics. 2020; 199(1-2): 355-361. doi: 10.1007/s10909-019-02313-4
16. Gao J, Mazin B, Daal M, et al. Power dependence of phase noise in microwave kinetic inductance detectors. SPIE Proceedings. 2006; 6275(1).
17. Gao J. The Physics of Superconducting Microwave Resonators [PhD thesis]. California Institute of Technology; 2008.
18. Noroozian O. Superconducting microwave resonator arrays for submillimeter/far-infrared imaging [PhD thesis]. California Institute of Technology; 2012.
19. Zobrist N, Daal M, Corbin JY, et al. Disk Resonator Design for Kinetic Inductance Detectors. Journal of Low Temperature Physics. 2018; 194(5-6): 394-403. doi: 10.1007/s10909-018-02125-y
20. Baselmans J. Kinetic Inductance Detectors. Journal of Low Temperature Physics. 2012; 167(3-4): 292-304. doi: 10.1007/s10909-011-0448-8
21. Doyle S, Mauskopf P, Naylon J, et al. Lumped Element Kinetic Inductance Detectors. Journal of Low Temperature Physics. 2008; 151(1-2): 530-536. doi: 10.1007/s10909-007-9685-2
22. Doyle S, Mauskopf P, Zhang J, et al. Optimisation of Lumped Element Kinetic Inductance Detectors for use in ground based mm and sub-mm arrays. AIP Conference Proceedings. 2009; 1185(1).
23. Verheul S. Ultra High Kinetic Inductance Detectors [Master’s thesis]. Delft University of Technology; 2019.
24. De Lucia M, Ulbricht G, Baldwin E, et al. Limitations to the energy resolution of single-photon sensitive microwave kinetic inductance detectors. AIP Advances. 2023; 13(12). doi: 10.1063/5.0168365
25. Cardani L, Colantoni I, Cruciani A, et al. Energy resolution and efficiency of phonon-mediated kinetic inductance detectors for light detection. Applied Physics Letters. 2015; 107(9). doi: 10.1063/1.4929977
26. Schlaerth J, Golwala S, Zmuidzinas J, et al. Sensitivity Optimization of Millimeter/Submillimeter MKID Camera Pixel Device Design. AIP Conference Proceedings. 2009; 1185(1).
27. Leduc HG, Bumble B, Day PK, et al. Titanium nitride films for ultrasensitive microresonator detectors. Applied Physics Letters. 2010; 97(10). doi: 10.1063/1.3480420
28. Mazin BA, Day PK, Zmuidzinas J, Leduc HG. Multiplexable kinetic inductance detectors. AIP Conference Proceedings. 2002; 605(1).
29. Baselmans J, Barends R, Hovenier JN, et al. High Q Niobium superconducting resonators for use as Kinetic Inductance sensing elements Proceedings of the European Interferometry Initiative Workshop organized in the context of the 20005 Joint European and National Astronomy Meeting “Distant Worlds”. Bulletin de la Société Royale des Sciences de Liège. 2005; 74(5-6): 491-497.
30. Doyle S, Dunscombe C, Mauskopf P, et al. Detection of Terahertz radiation using large Niobium detector arrays. In: Proceedings of the 16th International Symposium on Space Terahertz Technology.
31. Mazin BA, Bumble B, Day PK, et al. Position sensitive x-ray spectrophotometer using microwave kinetic inductance detectors. Applied Physics Letters. 2006; 89(22). doi: 10.1063/1.2390664
32. Barends R, Baselmans JJA, Hovenier JN, et al. Niobium and Tantalum High Q Resonators for Photon Detectors. IEEE Transactions on Applied Superconductivity. 2007; 17(2): 263-266. doi: 10.1109/tasc.2007.898541
33. Baselmans J, Yates SJC, Barends R, et al. Noise and Sensitivity of Aluminum Kinetic Inductance Detectors for Sub-mm Astronomy. Journal of Low Temperature Physics. 2008; 151(1-2): 524-529. doi: 10.1007/s10909-007-9684-3
34. Kumar S, Gao J, Zmuidzinas J, et al. Temperature dependence of the frequency and noise of superconducting coplanar waveguide resonators. Applied Physics Letters. 2008; 92(12).
35. Gao J, Daal M, Martinis JM, et al. A semiempirical model for two-level system noise in superconducting microresonators. Applied Physics Letters. 2008; 92(21). doi: 10.1063/1.2937855
36. Noroozian O, Gao J, Zmuidzinas J, et al. Two-level system noise reduction for Microwave Kinetic Inductance Detectors. AIP Conference Proceedings. 2009; 1185(1).
37. Czakon NG, Schlaerth JA, Day PK, et al. Optimization of MKID noise performance via readout technique for astronomical applications. SPIE Proceedings. 2010; 7441.
38. Yates SJC, Baselmans JJA, Endo A, et al. Photon noise limited radiation detection with lens-antenna coupled microwave kinetic inductance detectors. Applied Physics Letters. 2011; 99(7): 073505. doi: 10.1063/1.3624846
39. Shirokoff E, Barry PS, Bradford CM, et al. MKID development for SuperSpec: An on-chip, mm-wave, filter-bank spectrometer. SPIE Proceedings. 2012; 8452(1).
40. Janssen RMJ, Baselmans JJA, Endo A, et al. High optical efficiency and photon noise limited sensitivity of microwave kinetic inductance detectors using phase readout. Applied Physics Letters. 2013; 103(20): 203503. doi: 10.1063/1.4829657
41. Lowitz AE, Brown AD, Stevenson TR, et al. Design, fabrication, and testing of lumped element kinetic inductance detectors for 3 mm CMB Observations. SPIE Proceedings. 2014; 9153(1)
42. Araujo DC, Ade PAR, Bond JR, et al. A LEKID-based CMB instrument design for large-scale observations in Greenland. SPIE Proceedings. 2014; 9153(1).
43. Ji C. Design of antenna-coupled lumped-element titanium nitride KIDs for long-wavelength multi-band continuum imaging. California Institute of Technology; 2015.
44. McCarrick H, Abitbol MH, Ade PAR, et al. Development of dual-polarization LEKIDs for CMB observations. SPIE Proceedings. 2016; 9914(1).
45. Johnson BR, Flanigan D, Abitbol MH, et al. Polarization sensitive Multi-Chroic MKIDs. SPIE Proceedings. 2016; 9914(1).
46. Bueno J, Yurduseven O, Yates SJC, et al. Full characterisation of a background limited antenna coupled KID over an octave of bandwidth for THz radiation. Applied Physics Letters. 2017; 110(23). doi: 10.1063/1.4985060
47. Beldi S, Boussaha F, Hu J, et al. High Q-factor near infrared and visible Al2O3-based parallel-plate capacitor kinetic inductance detectors. Optics Express. 2019; 27(9): 13319. doi: 10.1364/oe.27.013319
48. Hornsby AL, Barry PS, Doyle SM, et al. Reducing the Susceptibility of Lumped-Element KIDs to Two-Level System Effects. Journal of Low Temperature Physics. 2020; 200(5-6): 239-246. doi: 10.1007/s10909-020-02501-7
49. Hailey-Dunsheath S, Janssen RMJ, Glenn J, et al. Kinetic inductance detectors for the Origins Space Telescope. Journal of Astronomical Telescopes, Instruments, and Systems. 2021; 7(01). doi: 10.1117/1.jatis.7.1.011015
50. Sueno Y, Honda S, Kutsuma H, et al. Characterization of two-level system noise for a microwave kinetic inductance detector comprising niobium film on a silicon substrate. Progress of Theoretical and Experimental Physics. 2022; 2022(3). doi: 10.1093/ptep/ptac023
51. Hood JC, Barry PS, Cecil T, et al. Testing Low-Loss Microstrip Materials with MKIDs for Microwave Applications. Journal of Low Temperature Physics. 2022; 209(5-6): 1189-1195. doi: 10.1007/s10909-022-02881-y
52. Pan Z, Dibert KR, Zhang J, et al. Noise Optimization for MKIDs With Different Design Geometries and Material Selections. IEEE Transactions on Applied Superconductivity. 2023; 33(5): 1-8. doi: 10.1109/tasc.2023.3250167
Refbacks
- There are currently no refbacks.
Copyright (c) 2024 Zahra Ehsani, Seyed Mohammad Hassan Javadzadeh, Mahboobeh Baghi Hedeshi
License URL: https://creativecommons.org/licenses/by/4.0/
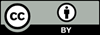
This site is licensed under a Creative Commons Attribution 4.0 International License (CC BY 4.0).
1.jpg)
Prof. Sivanesan Subramanian
Anna University, India
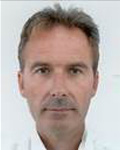
Prof. Pascal Lorenz
University of Haute Alsace, France
Dortmund University of Technology, Germany.
Interests: Mass spectrometry, Molecular Structural Analysis, Methodology; Application; Biological, Environmental and Food samples.