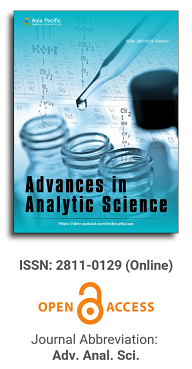
Asia Pacific Academy of Science Pte. Ltd. (APACSCI) specializes in international journal publishing. APACSCI adopts the open access publishing model and provides an important communication bridge for academic groups whose interest fields include engineering, technology, medicine, computer, mathematics, agriculture and forestry, and environment.
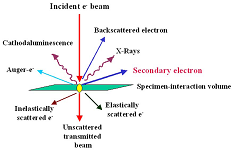
Natural and synthetic cavitands: Challenges in chemistry and pharmaceutical technology
Vol 3, Issue 1, 2022
Download PDF
Abstract
Supramolecular chemistry involves non-covalent interactions and specific molecular recognition of molecules/analytes by host molecules or supramolecules. These events are present in synthesis, catalysis, chiral separations, design of sensors, cell signaling processes and drug transport by carriers. The typical behavior of supramolecules is derived from their ability to build well-structured self-assembled and self-organized entities. Cavitands are a particular group of supramolecules possessing a cavity capable to include a variety of compounds thanks to host-guest non-covalent interactions developed among cavitands and analytes. Some typical cavitands are crown ethers, calixarenes, cucurbiturils, porphyrins and cyclodextrins. The two latter families are natural product cavitands that are generally considered models for molecular recognition of cations and organic and inorganic guest molecules, being attractive host molecules from the sustainability point of view. The natural cyclodextrins (α, β and γ-cd) are obtained with reasonable cost by enzymatic treatment of starch under adequate temperature conditions. They are profusely used in pharmaceutical, food and cosmetic industries due to their very low toxicity and side effects. This review is focused on the relevance andapplications of cyclodextrins in pharmaceuticaltechnology for their ability to increase solubility and stabilize drug molecules, thereby enhancing their bioavailability. The association of cyclodextrins with diverse nanostructured materials, i.e., carbon nanotubes, magnetic nanoparticles, silica and molecularly imprinted polymers, allows to synergize the properties of cyclodextrins and these nanostructured materials to reach highly specific molecular recognition of analytes. The exploitation of these benefits for analytical sample pre-treatment and chiral chromatographic separations are described. The use of cyclodextrins as mobile phases additives in hplc provides interesting results for green and sustainable chromatographic separations. Polymers incorporating cyclodextrins show exceptional adsorption properties for retaining toxic compounds and persistent organic pollutants from soils and water samples, allowing satisfactory recoveries of these environmental samples according to the stockholm convection principles.
Keywords
References
Refbacks
- There are currently no refbacks.
Copyright (c) 2022 María Antonia Martín Carmona

This work is licensed under a Creative Commons Attribution 4.0 International License.
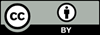
This site is licensed under a Creative Commons Attribution 4.0 International License (CC BY 4.0).
1.jpg)
Prof. Sivanesan Subramanian
Anna University, India