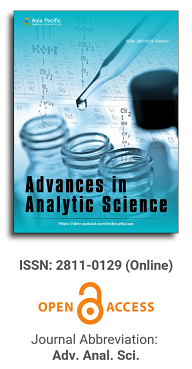
Asia Pacific Academy of Science Pte. Ltd. (APACSCI) specializes in international journal publishing. APACSCI adopts the open access publishing model and provides an important communication bridge for academic groups whose interest fields include engineering, technology, medicine, computer, mathematics, agriculture and forestry, and environment.
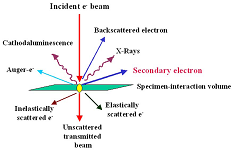
Advancements in analytical techniques for carbon nanomaterials
Vol 3, Issue 1, 2022
Download PDF
Abstract
With the mass production and application of carbon nanomaterials, effective detection and characterization methods in various media are crucial. This paper reviews commonly used techniques for analyzing carbon nanomaterials, starting with their separation and enrichment methods, including extraction and fractionation technologies. It then covers characterization techniques such as electron microscopy, spectroscopy, thermal analysis, electrochemical analysis, isotope labeling, imaging, fluorescence spectroscopy, laser-induced breakdown spectroscopy, mass spectrometry, scanning Raman microscopy, and quantitative analysis methods. The paper also introduces new carbon nanomaterials and specialized characterization methods, concluding with a discussion on future trends and prospects in the field.
Keywords
References
- Baptista FR, Belhout SA, Giordani S, et al. Recent developments in carbon nanomaterial sensors. Chemical Society Reviews. 2015; 44(13): 4433-4453. doi: 10.1039/c4cs00379a
- Li X, Ping J, Ying Y. Recent developments in carbon nanomaterial-enabled electrochemical sensors for nitrite detection. TrAC Trends in Analytical Chemistry. 2019; 113: 1-12. doi: 10.1016/j.trac.2019.01.008
- Wang J, Liu Q, Liang Y, et al. Recent progress in application of carbon nanomaterials in laser desorption/ionization mass spectrometry. Analytical and Bioanalytical Chemistry. 2016; 408(11): 2861-2873. doi: 10.1007/s00216-015- 9255-4
- Cai D, Mataraza JM, Qin ZH, et al. Highly efficient molecular delivery into mammalian cells using carbon nanotube spearing. Nature Methods. 2005; 2(6): 449-454. doi: 10.1038/nmeth761
- Baker SN, Baker GA. Luminescent Carbon Nanodots: Emergent Nanolights. Angewandte Chemie International Edition. 2010; 49(38): 6726-6744. doi: 10.1002/anie.200906623
- Karbasi S, Alizadeh ZM. Effects of multi-wall carbon nanotubes on structural and mechanical properties of poly(3- hydroxybutyrate)/chitosan electrospun scaffolds for cartilage tissue engineering. Bulletin of Materials Science. 2017; 40(6): 1247-1253. doi: 10.1007/s12034-017-1479-9
- Mao HY, Laurent S, Chen W, et al. Graphene: Promises, Facts, Opportunities, and Challenges in Nanomedicine. Chemical Reviews. 2013; 113(5): 3407-3424. doi: 10.1021/cr300335p
- Schipper ML, Nakayama-Ratchford N, Davis CR, et al. A pilot toxicology study of single-walled carbon nanotubes in a small sample of mice. Nature Nanotechnology. 2008; 3(4): 216-221. doi: 10.1038/nnano.2008.68
- Nel A, Xia T, Mädler L, et al. Toxic Potential of Materials at the Nanolevel. Science. 2006; 311(5761): 622-627. doi: 10.1126/science.1114397
- Colvin VL. The potential environmental impact of engineered nanomaterials. Nature Biotechnology. 2003; 21(10): 1166-1170. doi: 10.1038/nbt875
- Wang H, Yang ST, Cao A, et al. Quantification of Carbon Nanomaterials in Vivo. Accounts of Chemical Research. 2012; 46(3): 750-760. doi: 10.1021/ar200335j
- Ding W, Li L, Xiong K, et al. Shape Fixing via Salt Recrystallization: A Morphology-Controlled Approach To Convert Nanostructured Polymer to Carbon Nanomaterial as a Highly Active Catalyst for Oxygen Reduction Reaction. Journal of the American Chemical Society. 2015; 137(16): 5414-5420. doi: 10.1021/jacs.5b00292
- Huang X, Liu Q, Yao S, et al. Recent progress in the application of nanomaterials in the analysis of emerging chemical contaminants. Analytical Methods. 2017; 9(19): 2768-2783. doi: 10.1039/c7ay00859g
- TUZEN M, SOYLAK M. Multiwalled carbon nanotubes for speciation of chromium in environmental samples. Journal of Hazardous Materials. 2007; 147(1-2): 219-225. doi: 10.1016/j.jhazmat.2006.12.069
- Herrmann A, Diederich F, Thilgen C, et al. Chemistry of the Higher Fullerenes: Preparative isolation of C76 by HPLC and synthesis, separation, and characterization of Diels‐Alder monoadducts of C70 and C76. Helvetica Chimica Acta. 1994; 77(7): 1689-1706. doi: 10.1002/hlca.19940770703
- Hawkins JM, Lewis TA, Loren SD, et al. Organic chemistry of C60 (buckminsterfullerene): chromatography and osmylation. The Journal of Organic Chemistry. 1990; 55(26): 6250-6252. doi: 10.1021/jo00313a009
- Li J, Zhang M, Sun B, et al. Separation and purification of fullerenols for improved biocompatibility. Carbon. 2012; 50(2): 460-469. doi: 10.1016/j.carbon.2011.08.073
- Nadler M, Mahrholz T, Riedel U, et al. Preparation of colloidal carbon nanotube dispersions and their characterisation using a disc centrifuge. Carbon. 2008; 46(11): 1384-1392. doi: 10.1016/j.carbon.2008.05.024
- Cai D, Blair D, Dufort FJ, et al. Interaction between carbon nanotubes and mammalian cells: characterization by flow cytometry and application. Nanotechnology. 2008; 19(34): 345102. doi: 10.1088/0957-4484/19/34/345102
- Wilson NR, Pandey PA, Beanland R, et al. Graphene Oxide: Structural Analysis and Application as a Highly Transparent Support for Electron Microscopy. ACS Nano. 2009; 3(9): 2547-2556. doi: 10.1021/nn900694t
- Oshida K, Nakazawa T, Miyazaki T, Endo M. Application of image processing techniques for analysis of nano- and micro-spaces in carbon materials. Synthetic Metals. 2001; 125(2): 223. doi: 10.1016/S0379-6779(01)00535-5
- Yehliu K, Vander Wal RL, Boehman AL. Development of an HRTEM image analysis method to quantify carbon nanostructure. Combustion and Flame. 2011; 158(9): 1837-1851. doi: 10.1016/j.combustflame.2011.01.009
- Gaddam CK, Huang CH, Vander Wal RL. Quantification of nano-scale carbon structure by HRTEM and lattice fringe analysis. Pattern Recognition Letters. 2016; 76: 90-97. doi: 10.1016/j.patrec.2015.08.028
- Yang ZQ, Verbeeck J, Schryvers D, et al. TEM and Raman characterisation of diamond micro- and nanostructures in carbon spherules from upper soils. Diamond and Related Materials. 2008; 17(6): 937-943. doi: 10.1016/j.diamond.2008.01.104
- Guo D, Wei H, Chen X, et al. 3D hierarchical nitrogen-doped carbon nanoflower derived from chitosan for efficient electrocatalytic oxygen reduction and high performance lithium–sulfur batteries. Journal of Materials Chemistry A. 2017; 5(34): 18193-18206. doi: 10.1039/c7ta04728b
- Zhang H, Cao G, Wang Z, et al. Growth of Manganese Oxide Nanoflowers on Vertically-Aligned Carbon Nanotube Arrays for High-Rate Electrochemical Capacitive Energy Storage. Nano Letters. 2008; 8(9): 2664-2668. doi: 10.1021/nl800925j
- Ma X, Yuan B. Fabrication of carbon nanoflowers by plasma-enhanced chemical vapor deposition. Applied Surface Science. 2009; 255(18): 7846-7850. doi: 10.1016/j.apsusc.2009.03.061
- Kharisov B. A Review for Synthesis of Nanoflowers. Recent Patents on Nanotechnology. 2008; 2(3): 190-200. doi: 10.2174/187221008786369651
- Liu L, Zhou K, He P, et al. Synthesis and microwave absorption properties of carbon coil–carbon fiber hybrid materials. Materials Letters. 2013; 110: 76-79. doi: 10.1016/j.matlet.2013.07.131
- Alves JO, Zhuo C, Levendis YA, et al. Microstructural analysis of carbon nanomaterials produced from pyrolysis/combustion of Styrene-Butadiene-Rubber (SBR). Materials Research. 2011; 14(4): 499-504. doi: 10.1590/s1516-14392011005000078
- Bal S, Saha S. Comparison and analysis of physical properties of carbon nanomaterial-doped polymer composites. High Performance Polymers. 2014; 26(8): 953-960. doi: 10.1177/0954008314535823
- Farre M, Sanchis J, Barcelo D. Analysis and assessment of the occurrence, the fate and the behavior of nanomaterials in the environment. Trends in Analytical Chemistry. 2011; 30(3): 517. doi: 10.1016/j.trac.2010.11.014
- Jarrah NA, van Ommen JG, Lefferts L. Growing a carbon nano-fiber layer on a monolith support; effect of nickel loading and growth conditions. Journal of Materials Chemistry. 2004; 14(10): 1590. doi: 10.1039/b314585a
- Chinthaginjala JK, Bitter JH, Lefferts L. Thin layer of carbon-nano-fibers (CNFs) as catalyst support for fast mass transfer in hydrogenation of nitrite. Applied Catalysis A: General. 2010; 383(1-2): 24-32. doi: 10.1016/j.apcata.2010.05.013
- Keller D. Reconstruction of STM and AFM images distorted by finite-size tips. Surface Science. 1991; 253(1-3): 353. doi: 10.1016/0039-6028(91)90606-S
- Li QS, Lee GYH, Ong CN, et al. AFM indentation study of breast cancer cells. Biochemical and Biophysical Research Communications. 2008; 374(4): 609-613. doi: 10.1016/j.bbrc.2008.07.078
- Rief M, Gautel M, Oesterhelt F, et al. Reversible Unfolding of Individual Titin Immunoglobulin Domains by AFM. Science. 1997; 276(5315): 1109-1112. doi: 10.1126/science.276.5315.1109
- Brihuega I, Mallet P, González-Herrero H, et al. Unraveling the Intrinsic and Robust Nature of van Hove Singularities in Twisted Bilayer Graphene by Scanning Tunneling Microscopy and Theoretical Analysis. Physical Review Letters. 2012; 109(19). doi: 10.1103/physrevlett.109.196802
- Hagen A, Hertel T. Quantitative Analysis of Optical Spectra from Individual Single-Wall Carbon Nanotubes. Nano Letters. 2003; 3(3): 383-388. doi: 10.1021/nl020237o
- Huang X, Liu Q, Jiang G. Tuning the performance of graphene as a dual-ion-mode MALDI matrix by chemical functionalization and sample incubation. Talanta. 2019; 199: 532-540. doi: 10.1016/j.talanta.2019.03.010
- Liu Q, Cheng M, Wang J, et al. Graphene Oxide Nanoribbons: Improved Synthesis and Application in MALDI Mass Spectrometry. Chemistry – A European Journal. 2015; 21(14): 5594-5599. doi: 10.1002/chem.201406280
- Yu S, Jeong SG, Chung O, et al. Bio-based PCM/carbon nanomaterials composites with enhanced thermal conductivity. Solar Energy Materials and Solar Cells. 2014; 120: 549-554. doi: 10.1016/j.solmat.2013.09.037
- Röding M, Bradley SJ, Nydén M, et al. Fluorescence Lifetime Analysis of Graphene Quantum Dots. The Journal of Physical Chemistry C. 2014; 118(51): 30282-30290. doi: 10.1021/jp510436r
- Denk W, Strickler JH, Webb WW. Two-Photon Laser Scanning Fluorescence Microscopy. Science. 1990; 248(4951): 73-76. doi: 10.1126/science.2321027
- Esteves da Silva JCG, Gonçalves HMR. Analytical and bioanalytical applications of carbon dots. TrAC Trends in Analytical Chemistry. 2011; 30(8): 1327-1336. doi: 10.1016/j.trac.2011.04.009
- Wild E, Jones KC. Novel Method for the Direct Visualization of in Vivo Nanomaterials and Chemical Interactions in Plants. Environmental Science & Technology. 2009; 43(14): 5290-5294. doi: 10.1021/es900065h
- Heise HM, Kuckuk R, Ojha AK, et al. Characterisation of carbonaceous materials using Raman spectroscopy: a comparison of carbon nanotube filters, single‐ and multi‐walled nanotubes, graphitised porous carbon and graphite. Journal of Raman Spectroscopy. 2008; 40(3): 344-353. doi: 10.1002/jrs.2120
- Zhang N, Tong L, Zhang J. Graphene-Based Enhanced Raman Scattering toward Analytical Applications. Chemistry of Materials. 2016; 28(18): 6426-6435. doi: 10.1021/acs.chemmater.6b02925
- Liu Z, Li X, Tabakman SM, et al. Multiplexed Multicolor Raman Imaging of Live Cells with Isotopically Modified Single Walled Carbon Nanotubes. Journal of the American Chemical Society. 2008; 130(41): 13540-13541. doi: 10.1021/ja806242t
- Yang D, Velamakanni A, Bozoklu G, et al. Chemical analysis of graphene oxide films after heat and chemical treatments by X-ray photoelectron and Micro-Raman spectroscopy. Carbon. 2009; 47(1): 145-152. doi: 10.1016/j.carbon.2008.09.045
- Budde H, Coca-López N, Shi X, et al. Raman Radiation Patterns of Graphene. ACS Nano. 2015; 10(2): 1756-1763. doi: 10.1021/acsnano.5b06631
- Saito Y, Verma P, Masui K, et al. Nano‐scale analysis of graphene layers by tip‐enhanced near‐field Raman spectroscopy. Journal of Raman Spectroscopy. 2009; 40(10): 1434-1440. doi: 10.1002/jrs.2366
- Hu Q, Hirai M, Joshi RK, et al. Structural and electrical characteristics of nitrogen-doped nanocrystalline diamond films prepared by CVD. Journal of Physics D: Applied Physics. 2008; 42(2): 025301. doi: 10.1088/0022- 3727/42/2/025301
- Lin CT, Chen TH, Chin TS, et al. Quasi two-dimensional carbon nanobelts synthesized using a template method. Carbon. 2008; 46(5): 741-746. doi: 10.1016/j.carbon.2008.01.034
- Huang X, Liu Q, Fu J, et al. Screening of Toxic Chemicals in a Single Drop of Human Whole Blood Using Ordered Mesoporous Carbon as a Mass Spectrometry Probe. Analytical Chemistry. 2016; 88(7): 4107-4113. doi: 10.1021/acs.analchem.6b00444
- Baughman RH, Zakhidov AA, de Heer WA. Carbon Nanotubes--the Route Toward Applications. Science. 2002; 297(5582): 787-792. doi: 10.1126/science.1060928
- Jariwala D, Sangwan VK, Lauhon LJ, et al. Carbon nanomaterials for electronics, optoelectronics, photovoltaics, and sensing. Chem Soc Rev. 2013; 42(7): 2824-2860. doi: 10.1039/c2cs35335k
- Allen MJ, Tung VC, Kaner RB. Honeycomb Carbon: A Review of Graphene. Chemical Reviews. 2009; 110(1): 132- 145. doi: 10.1021/cr900070d
- Wang H, Wang J, Deng X, et al. Biodistribution of Carbon Single-Wall Carbon Nanotubes in Mice. Journal of Nanoscience and Nanotechnology. 2004; 4(8): 1019-1024. doi: 10.1166/jnn.2004.146
- Deng X, Jia G, Wang H, et al. Translocation and fate of multi-walled carbon nanotubes in vivo. Carbon. 2007; 45(7): 1419-1424. doi: 10.1016/j.carbon.2007.03.035
- Yang S, Guo W, Lin Y, et al. Biodistribution of Pristine Single-Walled Carbon Nanotubes In Vivo. The Journal of Physical Chemistry C. 2007; 111(48): 17761-17764. doi: 10.1021/jp070712c
- Ji ZQ, Sun H, Wang H, et al. Biodistribution and tumor uptake of C60(OH) x in mice. Journal of Nanoparticle Research. 2005; 8(1): 53-63. doi: 10.1007/s11051-005-9001-5
- Xu JY, Li QN, Li JG, et al. Biodistribution of 99mTc-C60(OH)x in Sprague–Dawley rats after intratracheal instillation. Carbon. 2007; 45(9): 1865-1870. doi: 10.1016/j.carbon.2007.04.030
- Tian L, Wang X, Cao L, et al. Preparation of Bulk 13C‐Enriched Graphene Materials. Journal of Nanomaterials. 2010; 2010(1). doi: 10.1155/2010/742167
- Saha SK, Chowdhury DP, Das SK, et al. Encapsulation of radioactive isotopes into C60 fullerene cage by recoil implantation technique. Nuclear Instruments and Methods in Physics Research Section B: Beam Interactions with Materials and Atoms. 2006; 243(2): 277-281. doi: 10.1016/j.nimb.2005.08.156
- Singh R, Pantarotto D, Lacerda L, et al. Tissue biodistribution and blood clearance rates of intravenously administered carbon nanotube radiotracers. Proceedings of the National Academy of Sciences. 2006; 103(9): 3357- 3362. doi: 10.1073/pnas.0509009103
- Li YG, Huang X, Liu RL, et al. Synthesis of [14C] quincetone. Journal of Radioanalytical and Nuclear Chemistry. 2005; 265(1): 127-131. doi: 10.1007/s10967-005-0802-x
- Bullard-Dillard R, Creek KE, Scrivens WA, et al. Tissue Sites of Uptake of14C-Labeled C60. Bioorganic Chemistry. 1996; 24(4): 376-385. doi: 10.1006/bioo.1996.0032
- Chen S, Xiong C, Liu H, et al. Mass spectrometry imaging reveals the sub-organ distribution of carbon nanomaterials. Nature Nanotechnology. 2015; 10(2): 176-182. doi: 10.1038/nnano.2014.282
- Bussy C, Cambedouzou J, Lanone S, et al. Carbon Nanotubes in Macrophages: Imaging and Chemical Analysis by X-ray Fluorescence Microscopy. Nano Letters. 2008; 8(9): 2659-2663. doi: 10.1021/nl800914m
- Bauhofer W, Kovacs JZ. A review and analysis of electrical percolation in carbon nanotube polymer composites. Composites Science and Technology. 2009; 69(10): 1486-1498. doi: 10.1016/j.compscitech.2008.06.018
- Wang Y, Jaiswal M, Lin M, et al. Electronic Properties of Nanodiamond Decorated Graphene. ACS Nano. 2012; 6(2): 1018-1025. doi: 10.1021/nn204362p
- Cioffi CT, Palkar A, Melin F, et al. A Carbon Nano‐Onion–Ferrocene Donor–Acceptor System: Synthesis, Characterization and Properties. Chemistry – A European Journal. 2009; 15(17): 4419-4427. doi: 10.1002/chem.200801818
- Zhang Q, Nghiem J, Silverberg GJ, et al. Semiquantitative Performance and Mechanism Evaluation of Carbon Nanomaterials as Cathode Coatings for Microbial Fouling Reduction. Liu SJ, ed. Applied and Environmental Microbiology. 2015; 81(14): 4744-4755. doi: 10.1128/aem.00582-15
- Doudrick K, Herckes P, Westerhoff P. Detection of Carbon Nanotubes in Environmental Matrices Using Programmed Thermal Analysis. Environmental Science & Technology. 2012; 46(22): 12246-12253. doi: 10.1021/es300804f
- Akbar NS. Heat transfer and carbon nano tubes analysis for the peristaltic flow in a diverging tube. Meccanica. 2014; 50(1): 39-47. doi: 10.1007/s11012-014-0067-y
- Bom D, Andrews R, Jacques D, et al. Thermogravimetric Analysis of the Oxidation of Multiwalled Carbon Nanotubes: Evidence for the Role of Defect Sites in Carbon Nanotube Chemistry. Nano Letters. 2002; 2(6): 615- 619. doi: 10.1021/nl020297u
- Kong BD, Paul S, Nardelli MB, et al. First-principles analysis of lattice thermal conductivity in monolayer and bilayer graphene. Physical Review B. 2009; 80(3). doi: 10.1103/physrevb.80.033406
- Goli P, Ning H, Li X, et al. Thermal Properties of Graphene–Copper–Graphene Heterogeneous Films. Nano Letters. 2014; 14(3): 1497-1503. doi: 10.1021/nl404719n
- Kosynkin DV, Higginbotham AL, Sinitskii A, et al. Longitudinal unzipping of carbon nanotubes to form graphene nanoribbons. Nature. 2009; 458(7240): 872-876. doi: 10.1038/nature07872
- Gholampour A, Valizadeh Kiamahalleh M, Tran DNH, et al. From Graphene Oxide to Reduced Graphene Oxide: Impact on the Physiochemical and Mechanical Properties of Graphene–Cement Composites. ACS Applied Materials & Interfaces. 2017; 9(49): 43275-43286. doi: 10.1021/acsami.7b16736
- Jin Z, McNicholas TP, Shih CJ, et al. Click Chemistry on Solution-Dispersed Graphene and Monolayer CVD Graphene. Chemistry of Materials. 2011; 23(14): 3362-3370. doi: 10.1021/cm201131v
- Lalwani G, Kwaczala AT, Kanakia S, et al. Fabrication and characterization of three-dimensional macroscopic all- carbon scaffolds. Carbon. 2013; 53: 90-100. doi: 10.1016/j.carbon.2012.10.035
- Heymann D, Korochantsev A, Nazarov MA, et al. Search for fullerenes C60and C70in Cretaceous–Tertiary boundary sediments from Turkmenistan, Kazakhstan, Georgia, Austria, and Denmark. Cretaceous Research. 1996; 17(3): 367-380. doi: 10.1006/cres.1996.0023
- Jehlička J, Frank O, Hamplová V, et al. Low extraction recovery of fullerene from carbonaceous geological materials spiked with C60. Carbon. 2005; 43(9): 1909-1917. doi: 10.1016/j.carbon.2005.02.040
- Englert JM, Vecera P, Knirsch KC, et al. Scanning-Raman-Microscopy for the Statistical Analysis of Covalently Functionalized Graphene. ACS Nano. 2013; 7(6): 5472-5482. doi: 10.1021/nn401481h
- Santa T, Yoshioka D, Homma H, et al. High-Performance Liquid Chromatography of Fullerence (C60) in Plasma Using Ultraviolet and Mass Spectrometric Detection. Biological and Pharmaceutical Bulletin. 1995; 18(9): 1171- 1174. doi: 10.1248/bpb.18.1171
- Isaacson CW, Usenko CY, Tanguay RL, et al. Quantification of Fullerenes by LC/ESI-MS and Its Application to in Vivo Toxicity Assays. Analytical Chemistry. 2007; 79(23): 9091-9097. doi: 10.1021/ac0712289
- Ku BK, Emery MS, Maynard AD, et al. In situstructure characterization of airborne carbon nanofibres by a tandem mobility–mass analysis. Nanotechnology. 2006; 17(14): 3613-3621. doi: 10.1088/0957-4484/17/14/042
- Mazzuckelli LF, Methner MM, Birch ME, et al. Identification and Characterization of Potential Sources of Worker Exposure to Carbon Nanofibers During Polymer Composite Laboratory Operations. Journal of Occupational and Environmental Hygiene. 2007; 4(12): D125-D130. doi: 10.1080/15459620701683871
- Tai JT, Lai YC, Yang JH, et al. Quantifying Nanosheet Graphene Oxide Using Electrospray-Differential Mobility Analysis. Analytical Chemistry. 2015; 87(7): 3884-3889. doi: 10.1021/ac504671k
- Wang X, Xu JB, Xie W, et al. Quantitative Analysis of Graphene Doping by Organic Molecular Charge Transfer. The Journal of Physical Chemistry C. 2011; 115(15): 7596-7602. doi: 10.1021/jp200386z
- Li CY, Chou TW. A structural mechanics approach for the analysis of carbon nanotubes. International Journal of Solids and Structures. 2003; 40(10): 2487. doi: 10.1016/S0020-7683(03)00056-8
- Behfar K, Naghdabadi R. Nanoscale vibrational analysis of a multi-layered graphene sheet embedded in an elastic medium. Composites Science and Technology. 2005; 65(7-8): 1159-1164. doi: 10.1016/j.compscitech.2004.11.011
- He XQ, Kitipornchai S, Liew KM. Resonance analysis of multi-layered graphene sheets used as nanoscale resonators. Nanotechnology. 2005; 16(10): 2086-2091. doi: 10.1088/0957-4484/16/10/018
- Potts JR, Shankar O, Du L, et al. Processing–Morphology–Property Relationships and Composite Theory Analysis of Reduced Graphene Oxide/Natural Rubber Nanocomposites. Macromolecules. 2012; 45(15): 6045-6055. doi: 10.1021/ma300706k
- Song M, Gong Y, Yang J, et al. Free vibration and buckling analyses of edge-cracked functionally graded multilayer graphene nanoplatelet-reinforced composite beams resting on an elastic foundation. Journal of Sound and Vibration. 2019; 458: 89-108. doi: 10.1016/j.jsv.2019.06.023
- Ouyang W, Xu Z, Jia S, et al. Multilayer-graphene reinforced 316L matrix composites preparation by laser deposited additive manufacturing: microstructure and mechanical property analysis. Materials Research Express. 2019; 6(9): 096557. doi: 10.1088/2053-1591/ab2f2e
- Cui JP, Zhao WS, Yin WY, et al. Signal Transmission Analysis of Multilayer Graphene Nano-Ribbon (MLGNR) Interconnects. IEEE Transactions on Electromagnetic Compatibility. 2012; 54(1): 126-132. doi: 10.1109/temc.2011.2172947
- Nasiri SH, Moravvej-Farshi MK, Faez R. Stability Analysis in Graphene Nanoribbon Interconnects. IEEE Electron Device Letters. 2010; 31(12): 1458-1460. doi: 10.1109/led.2010.2079312
- Huang SF, Terakura K, Ozaki T, et al. First-principles calculation of the electronic properties of graphene clusters doped with nitrogen and boron: Analysis of catalytic activity for the oxygen reduction reaction. Physical Review B. 2009; 80(23). doi: 10.1103/physrevb.80.235410
- Palacios JJ, Ynduráin F. Critical analysis of vacancy-induced magnetism in monolayer and bilayer graphene. Physical Review B. 2012; 85(24). doi: 10.1103/physrevb.85.245443
- Ekşiogˇlu B, Nadarajah A. Structural analysis of conical carbon nanofibers. Carbon. 2006; 44(2): 360-373. doi: 10.1016/j.carbon.2005.07.007
- Fakhrabadi MMS, Khani N, Omidvar R, et al. Investigation of elastic and buckling properties of carbon nanocones using molecular mechanics approach. Computational Materials Science. 2012; 61: 248-256. doi: 10.1016/j.commatsci.2012.04.029
Refbacks
- There are currently no refbacks.
Copyright (c) 2022 Xiu Huang, Qian Liu, Guibin Jiang

This work is licensed under a Creative Commons Attribution 4.0 International License.
This site is licensed under a Creative Commons Attribution 4.0 International License (CC BY 4.0).
1.jpg)
Prof. Sivanesan Subramanian
Anna University, India