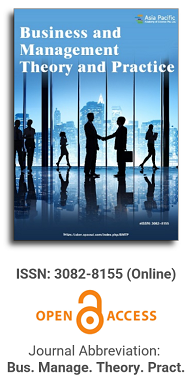
Asia Pacific Academy of Science Pte. Ltd. (APACSCI) specializes in international journal publishing. APACSCI adopts the open access publishing model and provides an important communication bridge for academic groups whose interest fields include engineering, technology, medicine, computer, mathematics, agriculture and forestry, and environment.
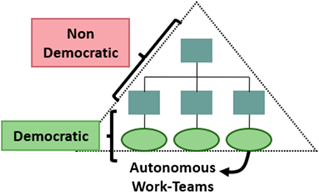
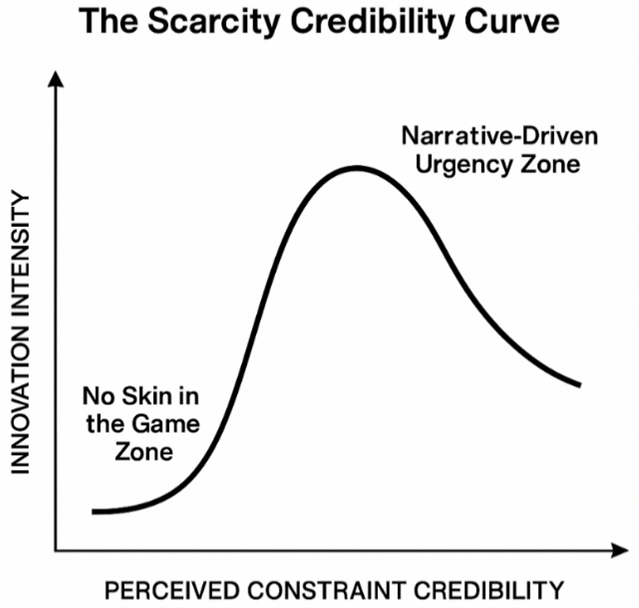
Application of Nano-Satellites constellation in the refinery mega projects implementation
Vol 1, Issue 1, 2024
Download PDF
Abstract
The purpose of this article is to provide scalable solutions based on new technologies in the field of refinery mega projects. Correcting defective structures and providing a new model and mechanism for completing projects in comprehensive and optimal manner may be conducted in terms of time, cost and quality of implementation. To this end, 156 effective factors in delaying Engineering Procurement and Construction (EPC) projects using field research are identified. Then, based on the knowledge and experience of the project managers and experts in each field, and analyzing their views using Analytical Hierarchy Process (AHP) method, the most effective factors in project deferrals are identified. Also, the main factors of excess costs in EPC are extracted. According to surveys, lake of the following factors lead to project deferrals: real-time communication, integrated information network platform, common and up-to-date databases, online status equipment and sensors, and up-to-date information and regular reports. In many cases, these delays and corresponding surplus costs are so high that they compromise the economic justification of the project in terms of inflation. To solve these problems, the proposed method captures the required information from each refinery segment using sensors and Radio Frequency Identification (RFID) tags mounted on the units and areas and client computers deployed in each refinery segment using Internet of Things (IoT) technology. The information is then transferred to central sites and data centers via Nano-satellite platforms. The received information is classified into databases and processed by software packages such as Enterprise Resource Planning (ERP) and finally are made accessible for other units. The study also includes the validation of Nano-satellite communications as the core of the proposed solution using Satellite Tool Kit (STK) software. Then the information is processed by business intelligence techniques and the required consultations are provided at three strategic levels: 1) for senior holding managers for decision making at tactical level, 2) for mid-level managers, and 3) at operational level for workshop managers to make the right decision at the right time. Achievements of implementing this solution includes systematic project execution, characterization of project components and executing company processes from start to the end while minimizing the project time and overhead costs, and selecting the best vendors and materials.
Keywords
References
- Naomi B. A Guide for Policy Makers and Practitioners. European Cooperation in Science and Technology. 2015.
- Javad K, Mohsen M, Faysal A. Investigating the Impact of the Structure of Iran’s Oil Contracts on Technology Transfer. Petroleum Business Review. 2023; 7(1): 105-122.
- Fatemeh H, Sepehr G, Mohammadreza A, et al. Technology planning system for the Iranian petroleum industry: Lessons learned from sanctions. Technological Forecasting and Social Change. 2017; 122: 170-178.
- Biousse R. Identification of Weaknesses and Problems of Domestic Companies in Implementation of EPC Projects in Oil and Gas Industry Using AHP Method. Young Oil Industry Experts Database; 2013.
- Tavakoli AR. Iran’s Leading Project Management (Based on Prince 2). Cultural and Artistic Institute of Intelligent Symbol Processing; 2014.
- Lai VS, Wong BK, & Cheung W. (2002). Group decision making in multiple criteria environment: A case using the AHP in software selection. European Journal of Operational Research. 2002.
- Lang TJ. Optimal low Earth orbit constellations for continuous global coverage. In: Proceedings of the Astrodynamics Conference; 1994.
- Durán O, Aguilo J. Computer-aided machine-tool selection based on a Fuzzy-AHP approach. Expert Systems with Applications. 2008; 34(3): 1787-1794. doi: 10.1016/j.eswa.2007.01.046
- Wei CC, Chien CF, Wang MJJ. An AHP-based approach to ERP system selection. International Journal of Production Economics. 2005; 96(1): 47-62. doi: 10.1016/j.ijpe.2004.03.004
- Celik M, Deha Er I, Ozok AF. Application of fuzzy extended AHP methodology on shipping registry selection: The case of Turkish maritime industry. Expert Systems with Applications. 2009; 36(1): 190-198. doi: 10.1016/j.eswa.2007.09.004
- Momeni M, Sharifi Salim AR. Multi-Characteristics Decision Making Models and Software. Tehran, Author Publishing; 2017.
- Sabzehpour M. Project Control. Tehran, Terme Publications; 2006.
- Pahlavani AS, Zarei B. Designing a methodology for identifying major project delays and presenting case study improvement strategies: Petrochemical Equipment Manufacturing Projects. In: Proceedings of the Second International Project Management Conference; Tehran; 2005.
- Braimah N. An investigation into the use of construction delay and disruption analysis methodologies [PhD thesis]. The University of Wolverhampton; 2008.
- James G, Zack Jr. Schedule Analysis is there agreement? Presentation; 2004.
- Irina G. Development of a robotic system for CubeSat Attitude Determination and Control System ground tests. HAL; 2018.
- Helvajian H, Janson S. Small Satellites: Past, Present, and Future. The Aerospace Press; 2008.
- Kaminskiy M. CubeSat Data Analysis Revision. National Aeronautics and Space Administration (NASA); 2015.
- Siegfried J. 25 Years of Small Satellite. Small Satellite Conference; 2011.
- Nieto-Peroy C, Emami MR. CubeSat Mission: From Design to Operation. Applied Sciences. 2019; 9(15): 3110. doi: 10.3390/app9153110
- Traussnig W. Design of a Communication and Navigation Subsystem for a CubeSat Mission. Karl Franzens University of Graz Graz, Austria; 2006.
- Michael Swartwout, The First One Hundred CubeSats, A Statistical LookP. Arks College of Engineering, Aviation and Technology, Saint Louis University, St. Louis, Missouri, USA; 2013.
- Coppa I, Woodgate P, Mohamed-Ghouse Zaffar. Global Outlook 2018. Spatial Information Industrya; 2018.
- The Australian Space Agency’s mission. Review of Australia’s Space Industry Capability. The Expert Reference Group for the Review; 2018.
- Scatteia L. Main trends and challenges in the space sector. PWC; 2019.
- Alfeeli B. Reignites country’s space ambitions with ground station and CubeSat projects. Satellite Pro Middle East; 2019.
- Cuadrado GG. Nanosatellites—The Tool for a New Economy of Space: Opening Space Frontiers to a Wider Audience. Journal of Aeronautics & Aerospace Engineering. 2017; 06(02). doi: 10.4172/2168-9792.1000192
- Li D. Hybrid Active and Passive Antenna Selection for Backscatter-Assisted MISO Systems. IEEE Transactions on Communications. 2020; 68(11): 7258-7269. doi: 10.1109/tcomm.2020.3014917
- Gregory F. Cyber Security Project, Job One for Space Force: Space Asset Cyber security. Harvard Kennedy School, Belfer Center, For Science and International Affairs; 2018.
- Abomhara M, Koien GM. Security and privacy in the Internet of Things: Current status and open issues. In: Proceedings of the 2014 International Conference on Privacy and Security in Mobile Systems (PRISMS); 2014.
- Jia L, Zhang Y, Yu J, et al. Design of Mega-Constellations for Global Uniform Coverage with Inter-Satellite Links. Aerospace. 2022; 9(5): 234. doi: 10.3390/aerospace9050234
- Wang Q, Hirata T. Relief Aircraft Dispatch Strategies Based on Different Levels of Information Sharing Systems. Aerospace. 2021; 8(10): 306. doi: 10.3390/aerospace8100306
- IDTechEx. Internet of Things (IoT): Business Opportunities 2015-2025. Available online: www.IDTechEx.com/research (accessed on 5 May 2024).
- Ilyas M. The Handbook of Ad Hoc Wireless Networks. Taylor and Francis Group; 2002.
- Elia V, Gnoni MG. Pervasiveness of RFID technology: A survey based on case studies analysis. International Journal of RF Technologies. 2013; 5(1-2): 41-61.
- Breland F, Offshore D. Maintenance history materializes with RFID. DrillingContractor; 2011.
- Khoo B. RFID—from Tracking to the Internet of Things: A Review of Developments. In: Proceedings of the 2010 IEEE/ACM Int’l Conference on Green Computing and Communications & Int’l Conference on Cyber, Physical and Social Computing; 2010.
- Sardroud JM, Limbachiya MC, Saremi AA. Ubiquitous Tracking and Locating of Construction Resource Using GIS and RFID. Engineering, Computer Science, Geography, Environmental Science ; 2010.
- Samuel G. The Internet of Things. The MIT Press Essential Knowledge Series; 2015.
- Atinegar Engineers Co. Available online: https://atinegar.com/blog/lan -setuping/- lan_diagram_image (accessed on 20 May 2024).
- Asmar S, Matousek S. Mars Cube One (MarCO)—The First Planetary Cube-Sat Mission (Mars Cube-Sat/Nano-Sat Workshop). NASA Jet Propulsion Laboratory; 2014.
- Andrew TK, John DB, John B, et al. INSPIRE: Interplanetary NanoSpacecraft Pathfinder in Relevant Environment. American Institute of Aeronautics and Astronautics; 2013.
- Sarda K, Grant C, Eagleson S., et al. Canadian Advanced Nano-space Experiment 2: On-Orbit Experiences with a Three-Kilogram Satellite. In: Proceedings of the IAA/USU Conference on Small Satellites; 2008.
- Akyildiz IF, Kak A. The Internet of Space Things/CubeSats: A ubiquitous cyber-physical system for the connected world. Computer Networks. 2019; 150: 134-149. doi: 10.1016/j.comnet.2018.12.017
- Rouhani S. Business Intelligence Evaluation Requirements in ERP. Modern Economics and Business; 2008.
- Ilčev SD. Global Mobile Satellite Distress System, Durban University of Technology (DUT) Durban South Africa. Springer; 2017.
- Williams, S., Williams. Capturing ROI through Business-Centric BI Development Methods, DM Review, August 2004. Information Systems Frontiers; 2004.
- Eugenia Stan-Dreamstime. Business Intelligence Pyramid Concept. Available online: https://www.dreamstime.com/stock-illustration-business-intelligence-pyramid-concept-using-design-processing-flow-steps-data-sources-etl-datawarehouse-olap-data-mining-data-image88703833 (accessed on 25 March 2024).
- Raul Amoros. Mapping Crude Oil Reserves Around the World. Available online: https://howmuch.net/articles/worlds-biggest-crude-oil-reserves-by-country (accessed on 25 March 2024).
- Wang Q, Li W, Yu Z, et al. An Overview of Emergency Communication Networks. Remote Sensing. 2023; 15(6): 1595. doi: 10.3390/rs15061595
Refbacks
- There are currently no refbacks.
Copyright (c) 2024 Author(s)
License URL: https://creativecommons.org/licenses/by/4.0/
This site is licensed under a Creative Commons Attribution 4.0 International License (CC BY 4.0).
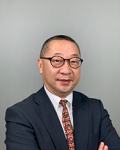
Macau University of Science and Technology, Macau